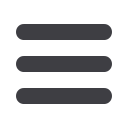
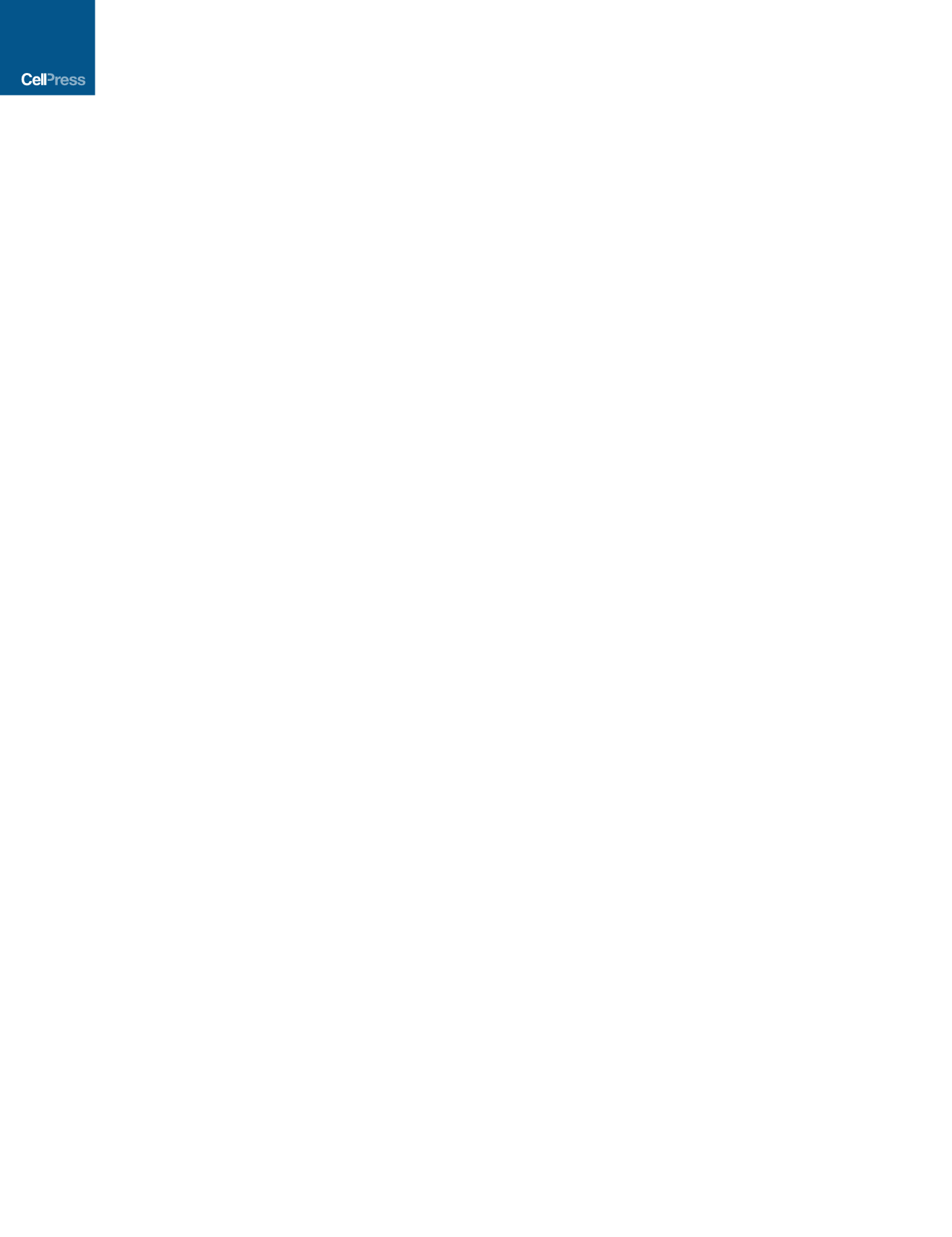
Our work demonstrates that the identification of specific mo-
tifs by carefully dissecting individual lncRNAs is critical for under-
standing overall lncRNA function and can explain why these
transcripts are overall lowly conserved at the sequence level.
Recently, in vivo DMS and SHAPE methods were developed to
directly probe RNA structure in living cells (Ding et al., 2014;
Rouskin et al., 2014; Spitale et al., 2013); however, the low abun-
dance of many lncRNAs makes it difficult probe their structures
in vivo, and the complex binding of proteins to RNAs can make
interpretation of probing signals more complicated. Thus, sec-
ondary structure maps of free RNA molecules are necessary to
facilitate a complete understanding of how these structures
contribute to lncRNAmodes of action under complex conditions.
Together, determination of lncRNA motifs using both in vitro and
in vivo probing results could be used to identify motif sequence
fingerprints and homologs across species through phylogenetic
sequence alignments and covariance analysis (Sanbonmatsu,
2016). Recent studies show that lncRNAs may undergo multiple
secondary structure conformations in vivo (Lu et al., 2016). Our
combined 3S and functional analyses confidently identify the
AGIL motif; however, we have not eliminated the possibility of
alternative folds. In some cases, RNA also forms higher-order
structures composed of tightly packed secondary structure ele-
ments (Leontis et al., 2006; Weeks, 2010). Thus, dissecting ter-
tiary structures of lncRNAs under physiological conditions also
represents an important area for future investigation. In vitro sec-
ondary structures of lncRNAs are also an important first step to-
ward crystallographic and cryoelectron microscopy (cryo-EM)
3D structures. Ultimately, studies aimed at mechanistic dissec-
tion of lncRNA structures are expected to facilitate a detailed un-
derstanding of how these transcripts contribute to fundamental
biological processes and open the door to exploiting RNA motifs
as biological and therapeutic tools.
EXPERIMENTAL PROCEDURES
Detailed experimental and analysis methods can be found in the Supplemental
Experimental Procedures.
Chemical Probing
SHAPE probing was performed using fast-acting 1M7 reagent (Deigan et al.,
2009), and DMS probing was performed as described (Tijerina et al., 2007).
ESC Lines and Growth Conditions
mESCs were cultured on irradiated mouse embryonic fibroblasts (MEFs) using
standard conditions as previously described (Wamstad et al., 2012). NKX2.5-
GFP mESCs (Hsiao et al., 2008) were used as WT ESCs in this study.
Generation of ESC Lines with CRISPR/Cas9
CRISPR/Cas9-mediated homology-directed repair or non-homologous end
joining was performed as described (Ran et al., 2013) using a bicistronic
expression vector expressing Cas9 and sgRNA (px330, Addgene #42230).
ESC Differentiation
EB formation and directed differentiation were performed as described (Klat-
tenhoff et al., 2013; Wamstad et al., 2012).
Immunostaining ESCs and Differentiated Cell Types
Cells were fixed and stained according to our previous studies (Klattenhoff
et al., 2013; Wamstad et al., 2012).
Flag-Tagged CNBP, HNRNPF, and SFSR9
Flag-tagged CNBP/HNRNPF/SFRS9 cassette was cloned into pEGIP
(Addgene #26777). Lentiviral production and ESC infection were performed
using protocols from the RNAi Consortium (Broad Institute).
RNA Immunoprecipitation
Cells were UV cross-linked and RNA immunoprecipitation was performed as
described (Jeon and Lee, 2011; Lai et al., 2013).
ProtoArray Processing and Analysis
In vitro RNA production and labeling followed by probing the ProtoArray
Human Protein Microarray v5.0 (Life Technologies cat# PAH0525101) were
performed as described (Siprashvili et al., 2012).
ACCESSION NUMBERS
The accession number for the ProtoArray raw data reported in this paper is
ArrayExpress
(http://www.ebi.ac.uk/arrayexpress): E-MTAB-4995.
SUPPLEMENTAL INFORMATION
Supplemental Information includes Supplemental Experimental Procedures,
seven figures, and two tables and can be found with this article online at
http://dx.doi.org/10.1016/j.molcel.2016.08.010.AUTHOR CONTRIBUTIONS
S.H. designed and performed chemical probing experiments; K.Y.S., S.H., and
I.V.N. analyzed the chemical probing data and determined the structure. Z.X.
and L.A.B. designed all other experiments and interpreted the results. Z.X.,
B.D., and A.A.G. performed these experiments. L.A.B. and Z.X. wrote the
manuscript.
ACKNOWLEDGMENTS
We thank members of the L.A.B. lab, David Bartel, Igor Ulitski, and Matt Talia-
ferro for insightful discussions and for critical evaluation of the manuscript. We
also thank Chikdu Shivalila for technical support in CRIPSR/Cas9 experi-
ments, Lionel Lam for help scanning the protein microarray, and the flow cy-
tometry core for technical support. K.Y.S., S.H., and I.V.N. were supported
by LANL-LDRD. A.A.G. was supported by the Sarnoff Cardiovascular
Research Foundation. This work was also supported in part by the Koch Insti-
tute Core grant (P30CA014051) and the NHLBI Bench to Bassinet Program
(U01HL098179) to L.A.B.
Received: March 17, 2016
Revised: June 21, 2016
Accepted: August 5, 2016
Published: September 8, 2016
REFERENCES
Aguilera, A., and Garcı´a-Muse, T. (2012). R loops: from transcription byprod-
ucts to threats to genome stability. Mol. Cell
46
, 115–124.
Armas, P., Nasif, S., and Calcaterra, N.B. (2008). Cellular nucleic acid binding
protein binds G-rich single-stranded nucleic acids and may function as a nu-
cleic acid chaperone. J. Cell. Biochem.
103
, 1013–1036.
Bindewald, E., Hayes, R., Yingling, Y.G., Kasprzak, W., and Shapiro, B.A.
(2008). RNAJunction: a database of RNA junctions and kissing loops for
three-dimensional structural analysis and nanodesign. Nucleic Acids Res.
36
, D392–D397.
Bondue, A., Lapouge, G., Paulissen, C., Semeraro, C., Iacovino, M., Kyba, M.,
and Blanpain, C. (2008). Mesp1 acts as a master regulator of multipotent car-
diovascular progenitor specification. Cell Stem Cell
3
, 69–84.
48
Molecular Cell
64
, 37–50, October 6, 2016