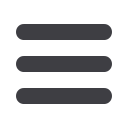
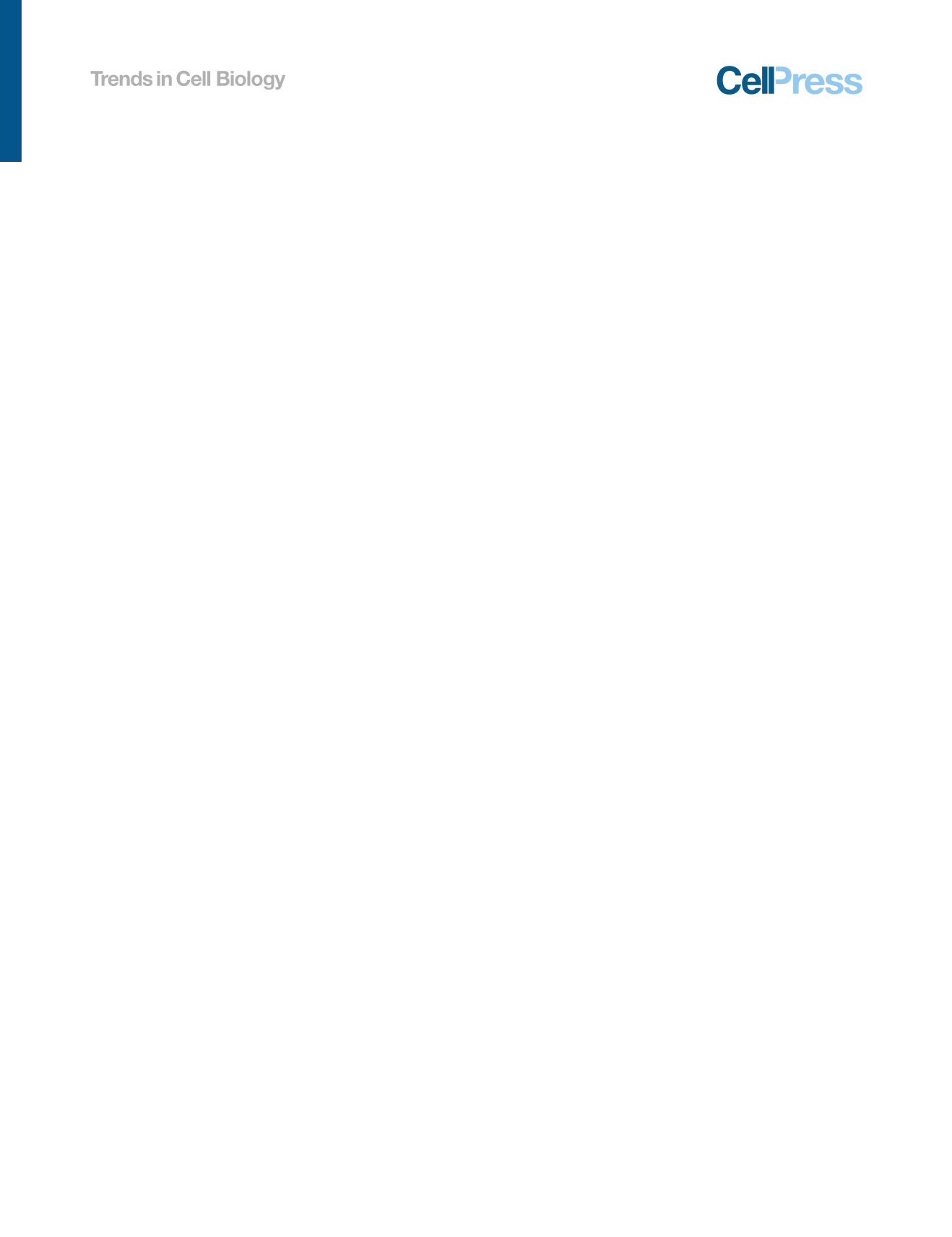
on-target activities comparable with wild-type Cas9 [113]. Combinations of these methods
could provide a route to its ultimate use for gene therapy.
As a powerful, yet versatile, gene-editing and regulation tool, CRISPR
[5_TD$DIFF]
/Cas9 technology is
already accelerating both research and therapeutics. We believe that its broad applications
in genomics research and cell biology research will greatly advance our knowledge of both basic
biology and diseases in the years to come.
[6_TD$DIFF]
Acknowledgments
F.W. acknowledges support from the Center of Synthetic Biology and Shanghai Institute of Rheumatology in Renji Hospital
af
fi
liated to Shanghai Jiaotong University School of Medicine, National Natural Science Foundation of China (No.
81502233), the Science and Technology Commission of Shanghai Municipality (No. 134119a8100) and the Young
Scienti
fi
c Research Project of Shanghai Municipal Health Bureau (No. 20134Y176). L.S.Q. acknowledges support from
the NIH Of
fi
ce of the Director (OD), National Institute of Dental & Craniofacial Research (NIDCR), and NIH Director's Early
Independence Award DP5 OD017887.
References
1.
Capecchi, M.R. (1989) Altering the genome by homologous
recombination.
Science
244, 1288
–
1292
2.
Rudin, N.
et al.
(1989) Genetic and physical analysis of double-
strand break repair and recombination in
Saccharomyces cer-
evisiae
.
Genetics
122, 519
–
534
3.
Bibikova, M.
et al.
(2001) Stimulation of homologous recombi-
nation through targeted cleavage by chimeric nucleases.
Mol.
Cell Biol.
21, 289
–
297
4.
Bibikova, M.
et al.
(2002) Targeted chromosomal cleavage and
mutagenesis in
Drosophila
using zinc-
fi
nger nucleases.
Genetics
161, 1169
–
1175
5.
Urnov, F.D.
et al.
(2005) Highly ef
fi
cient endogenous human gene
correction using designed zinc-
fi
nger nucleases.
Nature
435,
646
–
651
6.
Christian, M.
et al.
(2010) Targeting DNA double-strand breaks
with TAL effector nucleases.
Genetics
186, 757
–
761
7.
Gaj, T.
et al.
(2013) ZFN, TALEN, and CRISPR/Cas-based
methods for genome engineering.
Trends Biotechnol.
31,
397
–
405
8.
Wolfe, S.A.
et al.
(2000) DNA recognition by Cys2His2 zinc
fi
nger
proteins.
Annu. Rev. Biophys. Biomol. Struct.
29, 183
–
212
9.
Beerli, R.R. and Barbas, C.F., 3rd (2002) Engineering polydactyl
zinc-
fi
nger transcription factors.
Nat. Biotechnol.
20, 135
–
141
10. Konermann, S.
et al.
(2013) Optical control of mammalian
endogenous transcription and epigenetic states.
Nature
500,
472
–
476
11. Zhang, F.
et al.
(2011) Ef
fi
cient construction of sequence-speci
fi
c
TAL effectors for modulating mammalian transcription.
Nat. Bio-
technol.
29, 149
–
153
12. Maeder, M.L.
et al.
(2013) CRISPR RNA-guided activation of
endogenous human genes.
Nat. Methods
10, 977
–
979
13. Wiedenheft, B.
et al.
(2012) RNA-guided genetic silencing sys-
tems in bacteria and archaea.
Nature
482, 331
–
338
14. Marraf
fi
ni, L.A. and Sontheimer, E.J. (2010) CRISPR interference:
RNA-directed adaptive immunity in bacteria and archaea.
Nat.
Rev. Genet.
11, 181
–
190
15. Nakata, A.
et al.
(1982) Cloning of alkaline phosphatase isozyme
gene (iap) of
Escherichia coli
.
Gene
19, 313
–
319
16. Barrangou, R.
et al.
(2007) CRISPR provides acquired resistance
against viruses in prokaryotes.
Science
315, 1709
–
1712
17. Brouns, S.J.
et al.
(2008) Small CRISPR RNAs guide antiviral
defense in prokaryotes.
Science
321, 960
–
964
18. Jinek, M.
et al.
(2012) A programmable dual-RNA-guided DNA
endonuclease in adaptive bacterial immunity.
Science
337,
816
–
821
19. Deltcheva, E.
et al.
(2011) CRISPR RNA maturation by trans-
encoded small RNA and host factor RNase III.
Nature
471, 602
–
607
20. Sapranauskas, R.
et al.
(2011) The Streptococcus thermophilus
CRISPR/Cas system provides immunity in
Escherichia coli
.
Nucleic. Acids Res.
39, 9275
–
9282
21. Marraf
fi
ni, L.A. and Sontheimer, E.J. (2010) Self versus non-self
discrimination during CRISPR RNA-directed immunity.
Nature
463, 568
–
571
22. Cong, L.
et al.
(2013) Multiplex genome engineering using
CRISPR/Cas systems.
Science
339, 819
–
823
23. Mali, P.
et al.
(2013) RNA-guided human genome engineering via
Cas9.
Science
339, 823
–
826
24. Jinek, M.
et al.
(2013) RNA-programmed genome editing in
human cells.
Elife
2, e00471
25. Hwang, W.Y.
et al.
(2013) Ef
fi
cient genome editing in zebra
fi
sh
using a CRISPR-Cas system.
Nat. Biotechnol.
31, 227
–
229
26. Cho, S.W.
et al.
(2013) Targeted genome engineering in human
cells with the Cas9 RNA-guided endonuclease.
Nat. Biotechnol.
31, 230
–
232
27. Makarova, K.S.
et al.
(2015) An updated evolutionary classi
fi
ca-
tion of CRISPR-Cas systems.
Nat. Rev. Microbiol.
13, 722
–
736
28. Chylinski, K.
et al.
(2014) Classi
fi
cation and evolution of type II
CRISPR-Cas systems.
Nucleic. Acids Res.
42, 6091
–
6105
29. Gasiunas, G.
et al.
(2012) Cas9-crRNA ribonucleoprotein com-
plex mediates speci
fi
c DNA cleavage for adaptive immunity in
bacteria.
Proc. Natl. Acad. Sci. U.S.A.
109, E2579
–
E2586
30. Ran, F.A.
et al.
(2015) In vivo genome editing using
Staphylo-
coccus aureus
Cas9.
Nature
520, 186
–
191
31. Zhang, Y.
et al.
(2013) Processing-independent CRISPR RNAs
limit natural transformation in
Neisseria meningitidis
.
Mol. Cell
50,
488
–
503
32. Mali, P.
et al.
(2013) CAS9 transcriptional activators for target
speci
fi
city screening and paired nickases for cooperative
genome engineering.
Nat. Biotechnol.
31, 833
–
838
33. Sampson, T.R.
et al.
(2013) A CRISPR/Cas system mediates
bacterial innate immune evasion and virulence.
Nature
497, 254
–
257
34. Hirano, H.
et al.
(2016) Structure and engineering of
Francisella
novicida
Cas9.
Cell
164, 950
–
961
35. Hale, C.R.
et al.
(2009) RNA-guided RNA cleavage by a CRISPR
RNA-Cas protein complex.
Cell
139, 945
–
956
36. Hale, C.R.
et al.
(2012) Essential features and rational design of
CRISPR RNAs that function with the Cas RAMP module complex
to cleave RNAs.
Mol. Cell
45, 292
–
302
37. Zetsche, B.
et al.
(2015) Cpf1 is a single RNA-guided endonu-
clease of a class 2 CRISPR-Cas system.
Cell
163, 759
–
771
38. Yamano, T.
et al.
(2016) Crystal structure of Cpf1 in complex with
guide RNA and target DNA.
Cell
165, 949
–
962
39. Dong, D.
et al.
(2016) The crystal structure of Cpf1 in complex
with CRISPR RNA.
Nature
532, 522
–
526
886
Trends in Cell Biology, November 2016, Vol. 26, No. 11