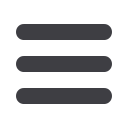
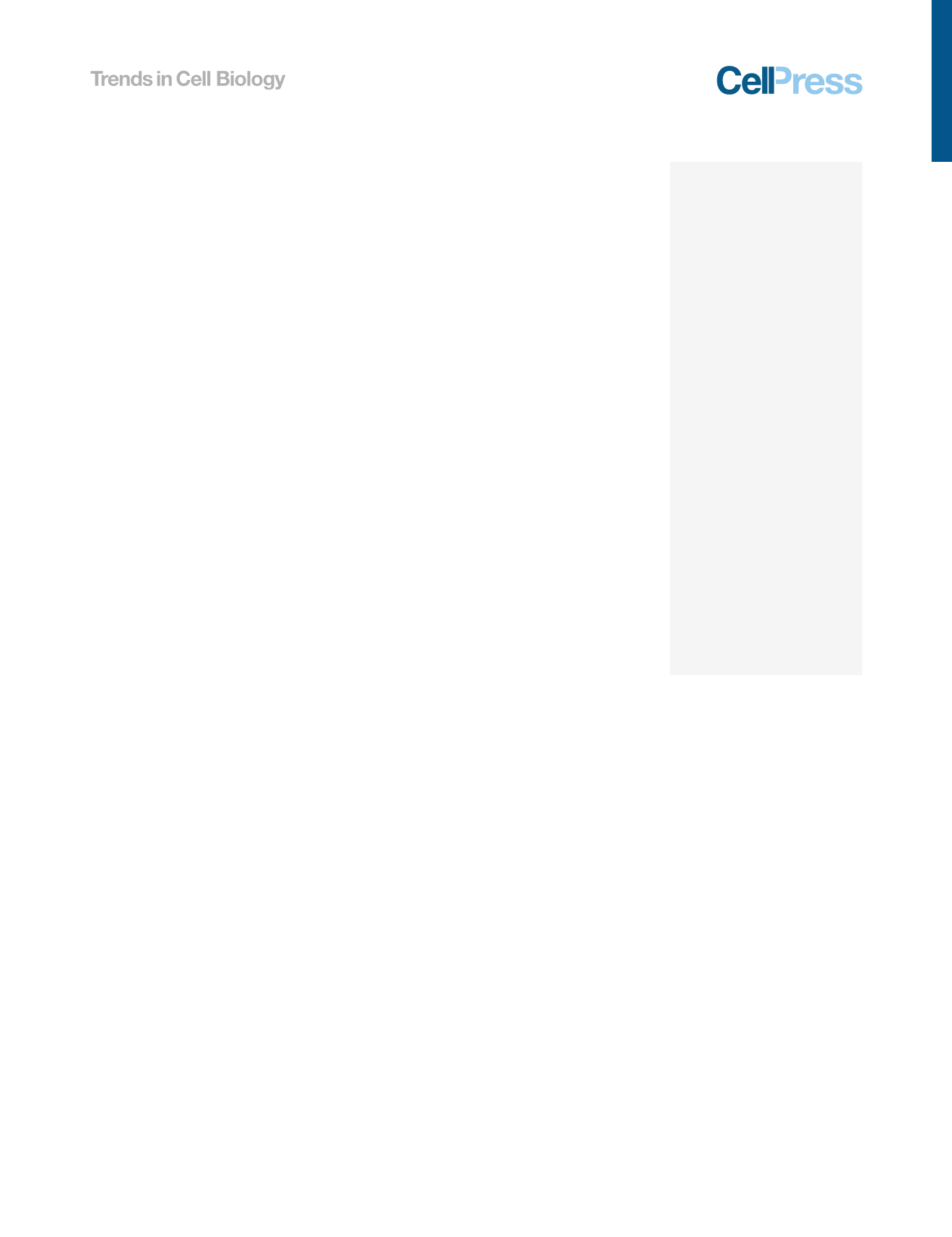
(GESTALT) [100]. This method uses CRISPR/Cas9 gene editing to generate a combinatorial
diversity of mutations that accumulate over cell divisions within a series of DNA barcodes. Via
deep sequencing, lineage relations between many cells can be inferred using patterns of the
edited barcodes. The approach was developed in both cell culture and zebra
fi
sh, by editing
synthetic arrays of approximately a dozen CRISPR/Cas9 target sites. The approach generated
thousands of unique edited barcodes in cell lines, which could then be sequenced from either
DNA or RNA. By injecting fertilized eggs with editing reagents that targeted a genomic barcode
with ten target sites, the authors observed the accumulation of hundreds to thousands of
uniquely edited barcodes per animal, and further inferred the lineage relations between ancestral
progenitors and organs based on mutation patterns. This proof-of-principle study showed that
combinatorial and cumulative genome editing is a powerful approach to record lineage infor-
mation in multicellular systems.
In another study, the type I-E CRISPR/Cas system of
E. coli
was harnessed to generate records
of speci
fi
c DNA sequences in bacterial genomes [101]. Unlike gene editing, the work was based
on the native adaptive immunity acquisition ability of CRISPR, because new spacer sequences
can be acquired and integrated stably into the CRISPR crRNA array. Using this feature, it was
demonstrated that the Cas1
–
Cas2 complex enables the recording of de
fi
ned sequences over
many days and in multiple modalities. The work elucidated fundamental aspects of the CRISPR
acquisition process. The recording system developed could be useful for applications that
require long histories of
in vivo
cellular activity to be traced.
While optimization of these methods is required for more robust performance, genome editing
and the unique features (i.e., adaptation) of the CRISPR system provide promising approaches
to record biological information and history in living cells and tissues. One can envision that these
tools may enable mapping of the complete cell lineage in multicellular organisms as well as linking
cell lineage information to molecular pro
fi
les (e.g., transcription, epigenetics, and proteomics),
such as those in single cells.
Concluding Remarks
The CRISPR/Cas9 technology has revolutionized cell biology research. The system is
versatile, enabling diverse types of genome engineering approach. While most of the work
has used Cas9-mediated knockout or dCas9-mediated repression and activation to study
gene function, we expect expansion of these tools to study the epigenome and 3D
chromosomal organization in greater detail in the future. Furthermore, studies have used
CRISPR to model complex genomic rearrangements
in vitro
and
in vivo
, which resulted in
breakthroughs in studying chromosomal translocations [102,103]. Most research has been
performed in cell lines, and future work related to the interrogation of cellular functions
should be carried out in primary cells derived from animals or humans or
in vivo
using
relevant animal models.
CRISPR/Cas9 is emerging as a major genome-manipulation tool for research and therapeutics,
yet there are challenges remaining to improve its speci
fi
city, ef
fi
ciency, and utility (see Outstand-
ing Questions). One major concern is the off-target effects, since Cas9 can tolerate mismatches
between sgRNA and target DNA [104
–
106]. Methods have been developed to pro
fi
le the off-
target effects, such as GUIDE-seq [107]. To improve speci
fi
city, several strategies have been
developed, including using paired nickase variants of Cas9 [32,42], paired dCas9-FokI nucle-
ases [108,109], truncated sgRNAs (17
–
18 base pairs) that are more sensitive to mismatches
[110], and controlling acting concentration of the Cas9/sgRNA complex [111]. Using structure-
guided protein-engineering approaches, two studies recently created
S. pyogenes
Cas9
variants with improved speci
fi
city [112,113]. For example, a high-
fi
delity variant of Cas9 har-
boring designed alterations showed reduced nonspeci
fi
c DNA contacts, while retaining robust
Outstanding Questions
How can the off-target effects of
CRISPR/Cas9 be avoided in mamma-
lian cells and whole organisms?
Can CRISPR/Cas9 technology be
developed to insert a large gene frag-
ment into the mammalian genome for
gene knock-in studies with similar ef
fi
-
ciency to that of gene knockout
studies?
Will CRISPR/Cas9 technology be able
to ef
fi
ciently modulate different types of
epigenetic modi
fi
cation? Can it control
the fate of synthetic epigenetic marks,
and whether they can be stably inher-
ited when cells proliferate?
Can CRISPR/Cas9-mediated genetic
screens be performed on nonprolifera-
tion-based phenotypes such as
differentiation?
Can CRISPR/Cas9 technology enable
more robust transgenic animal gener-
ation by deleting, mutating, and insert-
ing any gene of interest?
Beyond gene editing, how can
CRISPR/Cas9 be used to help advance
cell biology research?
Trends in Cell Biology, November 2016, Vol. 26, No. 11
885