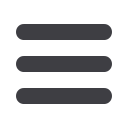
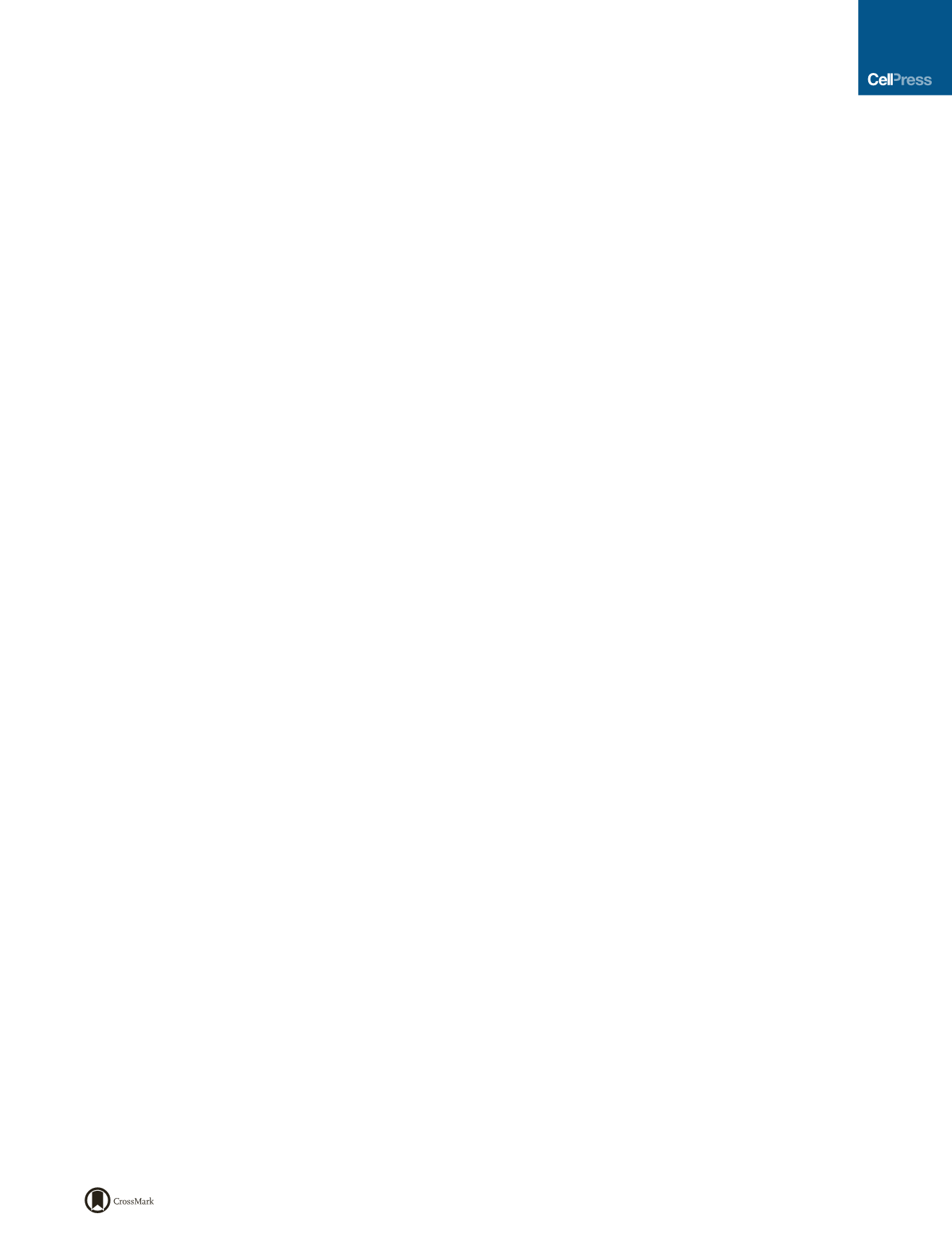
Molecular Cell
Article
A G-Rich Motif in the lncRNA
Braveheart
Interacts with a Zinc-Finger Transcription Factor
to Specify the Cardiovascular Lineage
Zhihong Xue,
1
Scott Hennelly,
2,3
Boryana Doyle,
4,5
Arune A. Gulati,
1
Irina V. Novikova,
2,6
Karissa Y. Sanbonmatsu,
2,3
and Laurie A. Boyer
1,7,8,
*
1
Department of Biology, Massachusetts Institute of Technology, Cambridge, MA 02139, USA
2
Los Alamos National Laboratory, Theoretical Biology and Biophysics Group, Los Alamos, NM 87545, USA
3
New Mexico Consortium, Los Alamos, NM 87544, USA
4
Undergraduate Research Opportunities Program
5
Department of Physics
Massachusetts Institute of Technology, Cambridge, MA 02139, USA
6
Pacific Northwest National Laboratory, Environmental Molecular Sciences Laboratory, Richmond, WA 99354, USA
7
Department of Biological Engineering, Massachusetts Institute of Technology, Cambridge, MA 02139, USA
8
Lead Contact
*Correspondence:
lboyer@mit.edu http://dx.doi.org/10.1016/j.molcel.2016.08.010SUMMARY
Long non-coding RNAs (lncRNAs) are an emerging
class of transcripts that can modulate gene expres-
sion; however, their mechanisms of action remain
poorly understood. Here, we experimentally deter-
mine the secondary structure of
Braveheart
(
Bvht
)
using chemical probing methods and show that
this 590 nt transcript has a modular fold. Using
CRISPR/Cas9-mediated editing of mouse embryonic
stem cells, we find that deletion of 11 nt in a 5
0
asym-
metric G-rich internal loop (AGIL) of
Bvht
(
bvht
dAGIL
)
dramatically impairs cardiomyocyte differentiation.
We demonstrate a specific interaction between
AGIL and cellular nucleic acid binding protein
(CNBP/ZNF9), a zinc-finger protein known to bind
single-stranded G-rich sequences. We further show
that CNBP deletion partially rescues the
bvht
dAGIL
mutant phenotype by restoring differentiation ca-
pacity. Together, our work shows that
Bvht
functions
with CNBP through a well-defined RNA motif to regu-
late cardiovascular lineage commitment, opening the
door for exploring broader roles of RNA structure in
development and disease.
INTRODUCTION
Long non-coding RNAs (lncRNAs) have emerged as important
regulators of development and disease. These transcripts are
typically >200 nt in length and are often polyadenylated, capped,
and alternatively spliced but lack coding potential (Ulitsky and
Bartel, 2013). Although biochemical and biophysical studies of
lncRNAs are in their early stages, proposed mechanisms of ac-
tion include chromatin scaffolding, Polycomb complex (PRC2)
recruitment to chromatin, mRNA decay, and decoys for proteins
and micro RNAs (miRNAs) (Geisler and Coller, 2013; Quinn and
Chang, 2016). Studies have highlighted diverse cellular roles
for lncRNAs across eukaryotes such as X chromosome inactiva-
tion, genomic imprinting, cell-cycle regulation, embryonic stem
cell (ESC) pluripotency, and lineage commitment (Flynn and
Chang, 2014; Lee and Bartolomei, 2013). In metazoans, there
is a growing number of lncRNAs that function in lineage commit-
ment and differentiation with key examples in the cardiovascular
system (Grote et al., 2013; Han et al., 2014; Klattenhoff et al.,
2013), including many that show differential expression in car-
diac disease (Fatica and Bozzoni, 2014; Rizki and Boyer,
2015). Thus, it remains a critical goal to understand how long
non-coding transcripts contribute to regulation of cell fate and
disease.
Comparative sequence analysis has facilitated RNA second-
ary structure predictions and has helped to reveal the functions
of ribonuclease P and riboswitches (Gutell et al., 2002; Mian,
1997; Parsch et al., 2000). These structural predictions are also
experimentally supported by chemical probing methods (e.g., in-
line, SHAPE, DMS), NMR, and X-ray crystallography (Mondra-
go´ n, 2013; Noller, 1984; Serganov and Patel, 2007). In contrast,
predicting lncRNA secondary structure has been more compli-
cated because these transcripts appear to be rapidly evolving
and generally display low sequence conservation (Ponting
et al., 2009). Recently, chemical probing methods have been ex-
ploited for studying lncRNA secondary structure. For example,
selective 2
0
hydroxyl acylation analyzed by primer extension
(SHAPE) probing of in vitro transcripts showed that the lncRNAs
SRA
and
HOTAIR
display a complex structural organization that
comprises a variety of elements comparable to well-folded RNAs
like group II introns and ribosomal RNAs (Novikova et al., 2012;
Somarowthu et al., 2015). Genome-wide probing of RNA sec-
ondary structure using dimethylsulfate sequencing (DMS-seq)
or in vivo click SHAPE sequencing (icSHAPE-seq) has also
been performed in living cells, revealing active unfolding of
mRNA structures, suggesting that RNA structures contribute to
Molecular Cell
64
, 37–50, October 6, 2016
ª
2016 Elsevier Inc.
37