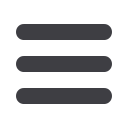
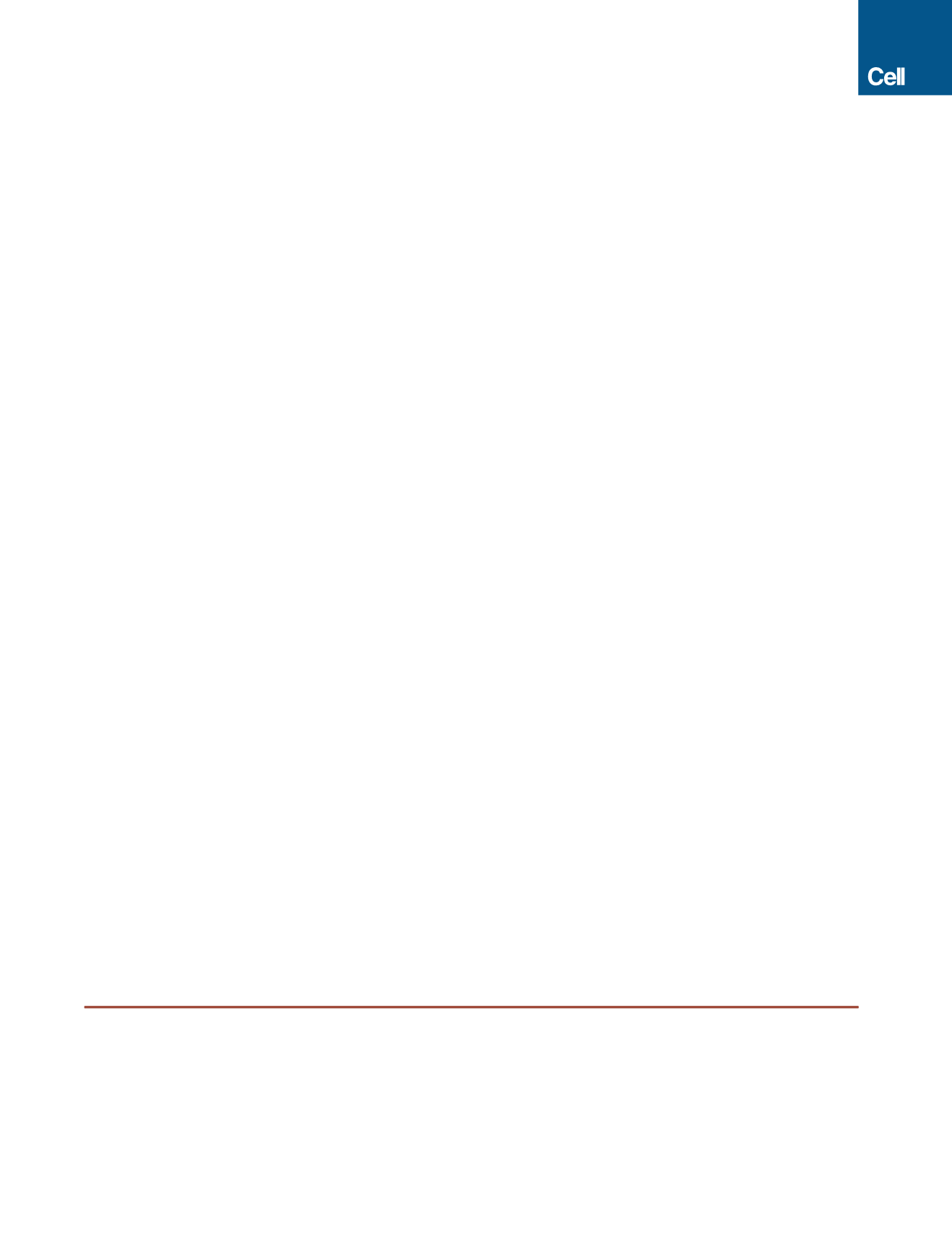
These experiments are labor intensive and time consuming.
Moreover, only 25% of blastocysts derived from genetic
crosses are homozygous mutants, posing a limitation for efficient
complementation. CRISPR-Cas9 mediated zygote genome edit-
ing offers a faster and more efficient one-step process for gener-
ating mice carrying homozygous mutations, thereby providing a
robust interspecies blastocyst complementation platform. Addi-
tionally, the multiplexing capability of CRISPR-Cas9 (Cong et al.,
2013; Yang et al., 2015) could potentially be harnessed for multi-
lineage complementation. For example, in the case of the
pancreas, one might hope to eliminate both the pancreatic pa-
renchyma and vasculature of the host to generate a more com-
plete xenogeneic pancreas. Despite the advantages, there are
several technical limitations of the CRISPR-Cas9 blastocyst
complementation system that need to be overcome before un-
locking its full potential. First, gene inactivation relies on the
error-prone, non-homologous end joining (NHEJ) pathway,
which is often unpredictable. In-frame mutations and mosaicism
are among the factors that may affect outcomes. A more predict-
able targeted gene inactivation strategy that utilizes homologous
recombination (HR) is still inefficient in the zygote. Second, each
embryo must be injected twice when using this system and em-
bryosmust be cultured in vitro for several days before ET, thereby
compromising embryo quality. Technical advancements that
include a more robust gene-disruption strategy (e.g., targeted
generation of frameshift mutations via homology independent
targeted integration [Suzuki et al., 2016]), alternative CRISPR/
Cas9 delivery methods, and improved culture conditions for
manipulated embryos will likely help improve and optimize the
generation of organogenesis-disabled hosts.
We observed a slower clearance of an intraperitoneally in-
jected glucose load for
Pdx1
/
than
Pdx1
+
/
rat-mouse chi-
meras, while both were slower than wild-type mouse controls
(Figure S2C). While this result may seem to contradict a previ-
ous report (Kobayashi et al., 2010), the discrepancy is likely
due to the development of autoimmune type inflammation that
is often observed in adult rat-mouse (chimeras made by injec-
tion of rat PSCs into mouse blastocyst, data not shown)
(>7 months, this study) and mouse-rat chimeras (chimeras
made by injection of mouse PSCs into rat blastocyst; H. Nakau-
chi, personal communication), which is less evident in young
chimeras ( 8 weeks; Kobayashi et al. 2010). Interestingly
though, we did observe a similarly slower clearance of glucose
load in wild-type rats, although the initial spike was much lower
in rats compared to mice or chimeras (Figure S2C). Thus, the rat
cellular origin might also have played a role in the different GTT
responses observed.
Rodent ESCs/iPSCs, considered as the gold standard cells for
defining naive pluripotency, can robustly contribute to intra- and
inter-species chimeras within rodent species. These and other
results have led to the assumption that naive PSCs are the cells
of choice when attempting to generate interspecies chimeras
involving more disparate species. Here, we show that rodent
PSCs fail to contribute to chimera formation when injected into
pig blastocysts. This highlights the importance of other contrib-
uting factors underlying interspecies chimerism that may
include, but not limited to, species-specific differences in
epiblast and trophectoderm development, developmental ki-
netics, and maternal microenvironment.
To date, and taking into consideration all published studies
that have used the mouse as the host species, it is probably
appropriate to conclude that interspecies chimera formation
involving hPSCs is inefficient (De Los Angeles et al., 2015). It
has been argued that this apparent inefficiency results from spe-
cies-specific differences between human and mouse embryo-
genesis. Therefore, studies utilizing other animal hosts would
help address this important question. Here we focused on two
species, pig and cattle, from a more diverse clade of mammals
and found that naive and intermediate, but not primed, hiPSCs
could robustly incorporate into pre-implantation host ICMs.
Following ET, we observed, in general and similar to the mouse
studies, low chimera forming efficiencies for all hiPSCs tested.
Interestingly, injected hiPSCs seemed to negatively affect
normal pig development as evidenced by the high proportion
of growth retarded embryos. Nonetheless, we observed that
FAC-hiPSCs, a putative intermediate PSC type between naive
and primed pluripotent states, displayed a higher level of chime-
rism in post-implantation pig embryos. IHC analyses revealed
that FAC-hiPSCs integrated and subsequently differentiated in
host pig embryos (as shown by the expression of different line-
age markers, and the lack of expression of the pluripotency
marker OCT4). Whether the degree of chimerism conferred by
FAC-hiPSCs could be sufficient for eliciting a successful inter-
species human-pig blastocyst complementation, as demon-
strated herein between rats and mice, remains to be demon-
strated. Studies and approaches to improve the efficiency and
level of hPSC interspecies chimerism (Wu et al., 2016), such as
matching developmental timing, providing a selective advantage
for donor hPSCs, generating diverse hPSCs with a higher
chimeric potential and selecting a species evolutionarily closer
to humans, among others parameters, will be needed.
The procedures and observations reported here on the capa-
bility of human pluripotent stem cells to integrate and differentiate
in a ungulate embryo, albeit at a low level and efficiency, when
Figure 6. Chimeric Contribution of hiPSCs to Post-implantation Pig Embryos
(A) Representative bright field (left top) fluorescence (left bottom and middle) and immunofluorescence (right) images of GFP-labeled FAC-hiPSCs derivatives in a
normal size day 28 pig embryo (FAC #1). Scale bar, 100
m
m.
(B) Representative immunofluorescence images showing chimeric contribution and differentiation of FAC-hiPSCs in a normal size, day 28 pig embryo (FAC #1).
FAC-hiPSC derivatives are visualized by antibodies against GFP (top), TUJ1, SMA, CK8 and HuNu (middle). (Bottom) Merged images with DAPI. Insets are higher
magnification images of boxed regions. Scale bar, 100
m
m.
(C) Representative gel images showing genomic PCR analyses of pig embryos derived from blastocyst injection of 2iLD-iPSCs (surrogates #8164 and #20749)
and FAC-hiPSCs (surrogates #9159 and #18771) using a human specific Alu primer. A pig specific primer Cyt b was used for loading control. nc, negative control
with no genomic DNA loaded. pc, positive controls with human cells. Pig 1D, 1G, and 1I, pig controls. ID, surrogate and pig embryos.
See also Figure S5 and Table S2.
Cell
168
, 473–486, January 26, 2017
483