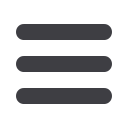
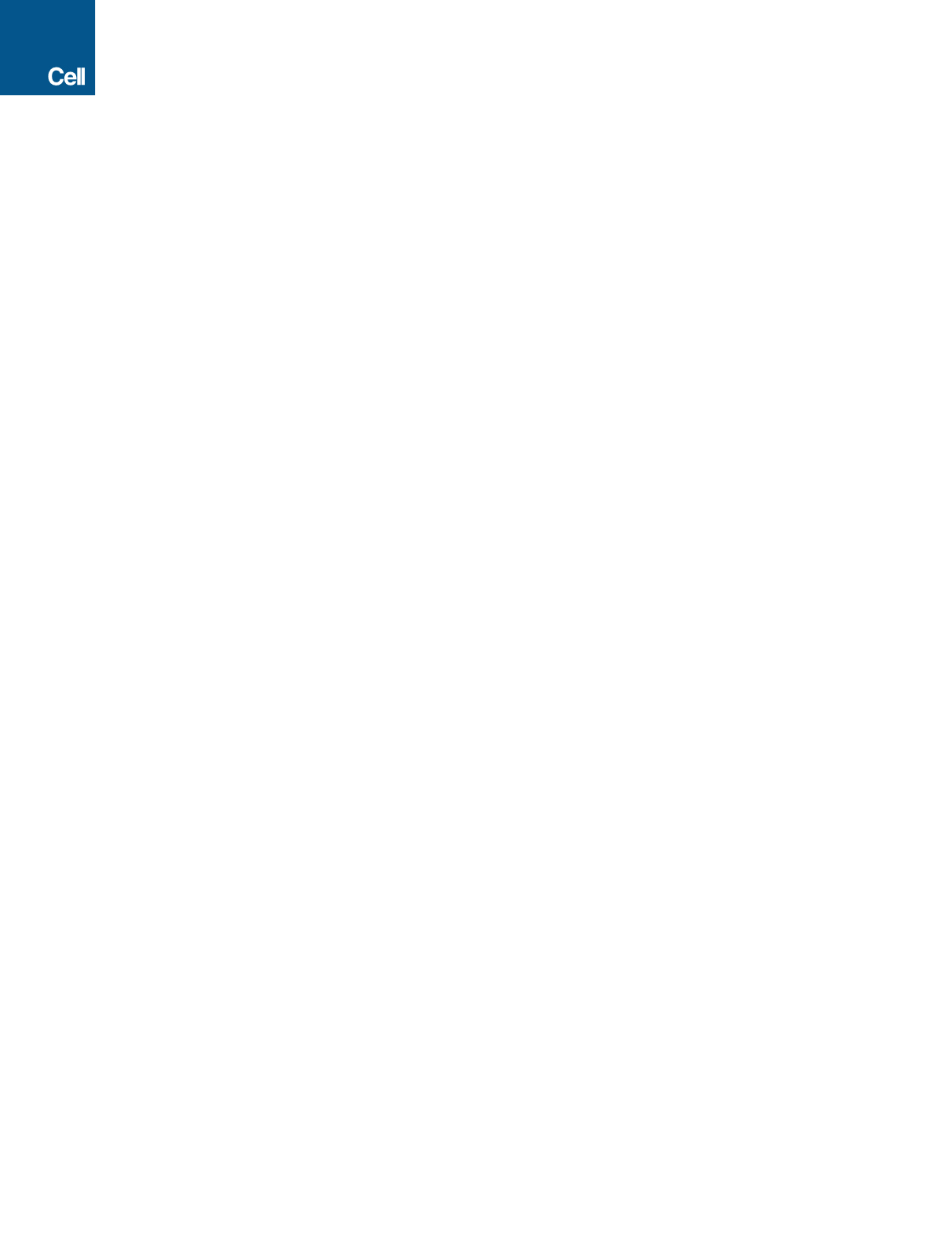
marker SOX2. These results indicate that both naive and inter-
mediate hiPSCs seem to perform better when injected into cattle
than pig blastocysts. This suggests a different in vivo blastocyst
environment in pig and cattle, with the cattle blastocysts
providing an environment that is more permissive for hiPSC inte-
gration and survival.
Chimeric Contribution of hiPSCs to Post-implantation
Pig Embryos
Although ICM incorporation of hiPSCs is the necessary first step
to contribute to the embryo proper of host animals, it has limited
predictive value for post-implantation chimera formation, as
other factors are involved. Next, we investigated if any of the
naive and intermediate hiPSCs that we generated, which
showed robust ICM incorporation in pre-implantation blasto-
cysts, could contribute to post-implantation development
following ET. The pig has certain advantages over cattle for ex-
periments involving post-implantation embryos, as they are a
polytocus species, and are commonly used as a translational
model given their similarities to humans concerning organ phys-
iology, size, and anatomy. We thus chose the pig for these ex-
periments. Since there was little to no contribution of primed
hiPSCs, even at the pre-implantation blastocyst stage, we
excluded these cells from the ET experiments. Pig embryos
were derived in vivo or through parthenogenesis. A total of 167
embryo donors were used in this study, from which we collected
1,298 zygotes, 1,004 two-cell embryos and 91 morulae (Table
S5). Embryos were cultured in vitro until they reached the blasto-
cyst stage (Figures S4AA and S4B). Overall, 2,181 good quality
blastocysts with a well-defined ICM were selected for subse-
quent blastocyst injections, of which 1,052 were derived from zy-
gotes, 897 from two-cell embryos, 91 from morulae, and 141
from parthenogenetic activation (Table S5). We injected 3-10
hiPSCs into the blastocoel of each of these blastocysts (Figures
5A, S4A, and S4C; Table S6). After in vitro embryo culture, a total
of 2,075 embryos (1,466 for hiPSCs; Table S6; 477 for rodent
PSCs; Table S3) that retained good quality were transferred to
surrogate sows. A total of 41 surrogate sows received 30–50 em-
bryos each, resulting in 18 pregnancies (Table S6). Collection of
embryos between day 21-28 of development resulted in the har-
vesting of 186 embryos: 43 from 2iLD-hiPSCs, 64 from FAC-
hiPSCs, 39 from 4i-hiPSCs, and 40 from NHSM-hiPSCs (Figures
5B, S4A, S4D, and S4F). In addition, 17 control embryos were
collected from an artificially inseminated sow (Figure 5B).
Following evaluating the developmental status of the obtained
embryos, more than half showed retarded growth and were
smaller than control embryos (Figures 5B and S4B), as was
seen when pig blastocysts were injected with rodent PSCs (Fig-
ure 3B). Among different hiPSCs, embryos injected with FAC-
hiPSCs were more frequently found to be normal size (Figure 5C).
From the recovered embryos, and based on fluorescence imag-
ing (GFP for 2iLD-hiPSCs and FAC-hiPSCs; hKO for 4i-hiPSCs
and NHSM-hiPSCs), we observed positive fluorescence signal
(FO+) in 67 embryos among which 17 showed a normal size
and morphology, whereas the rest were morphologically under-
developed (Figures 5B). In contrast, among fluorescence nega-
tive embryos we found the majority (82/119) appeared normal
size (Figure 5E), suggesting contribution of hiPSCs might have
interfered with normal pig development. Closer examination of
the underdeveloped embryos revealed that 50 out of 87 were
FO+ (Figures 5B). Among all the FO+ embryos the distribution
of normal size versus growth retarded embryos for each cell lines
was: 3:19 for 2iLD-hiPSCs, 7:14 for FAC-hiPSCs, 2:12 for 4i-
hiPSCs, and 5:5 for NHSM-hiPSCs (Figure 5D). Among normal
size embryos we found 3/13 from 2iLD-hiPSCs, 7/47 from
FAC-hiPSCs, 2/14 from 4i-hiPSCs, and 5/25 from NHSM-
hiPSCs that were FO+ (Figure 5B). All normal size FO+ embryos
derived from 2iLD-hiPSCs, 4i-hiPSCs, or NHSM-hiPSCs showed
a very limited fluorescence signal (Figure S5A). In contrast,
normal size FO+ FAC-hiPSC-derived embryos typically ex-
hibited a more robust fluorescence signal (Figures 6A and S5A).
Detecting fluorescence signal alone is insufficient to claim
chimeric contribution of donor hiPSCs to these embryos, as
auto-fluorescence from certain tissues and apoptotic cells can
yield false positives, especially when chimerism is low. We
thus sectioned all normal size embryos deemed positive based
on the presence of fluorescence signal and subjected them to
IHC analyses with antibodies detecting GFP or hKO. For 2iLD-
hiPSC-, 4i-hiPSC-, and NHSM-hiPSC-derived embryos, in
agreement with fluorescence signals observed in whole-embryo
analysis, we detected only a few hKO- or GFP-positive cells in
limited number of sections (Figure S5A). This precluded us
from conducting further IHC analysis using lineage markers.
For FAC-hiPSC-derived embryos, we confirmed via IHC analysis
(using an anti-GFP antibody) that they contained more human
cells (Figures 6A, S5A, and S5B). We then stained additional sec-
tions using antibodies against TUJ1, EPCAM, SMA, CK8, and
HNF3
b
(Figures 6B and S5C) and observed differentiation of
FAC-hiPSCs into different cell lineages. In addition, these cells
were found negative for OCT4, a pluripotency marker (data not
shown). Moreover, the presence of human cells was further veri-
fied with a human-specific HuNu antibody staining (Figure 6B)
and a sensitive genomic PCR assay using a human specific
Alu
sequence primer (Figure 6C; Table S2). Together, these re-
sults indicate that naive hiPSCs injected into pig blastocysts inef-
ficiently contribute to chimera formation, and are only rarely
detected in post-implantation pig embryos. An intermediate
hPSC type (FAC-hiPSCs) showed better chimeric contribution
and differentiated to several cell types in post-implantation
human-pig chimeric embryos. It should be noted that the
levels of chimerism from all hiPSCs, including the FAC-hiPSCs,
in pig embryos were much lower when compare to rat-mouse
chimeras (Figures 1C, 1E, S1A, and 1B), which may reflect the
larger evolutionary distance between human-pig than between
rat-mouse.
DISCUSSION
Our study confirms that live rat-mouse chimeras with extensive
contribution from naive rat PSCs can be generated. This is in
contrast to earlier work in which rat ICMs were injected into
mouse blastocysts (Gardner and Johnson, 1973). One possible
explanation for this discrepancy is that cultured PSCs acquire
artificial features that make themmore proliferative and/or better
able to survive than embryonic ICM cells, which in turn leads to
their more robust xeno-engraftment capability in a mouse host.
480
Cell
168
, 473–486, January 26, 2017