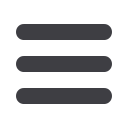
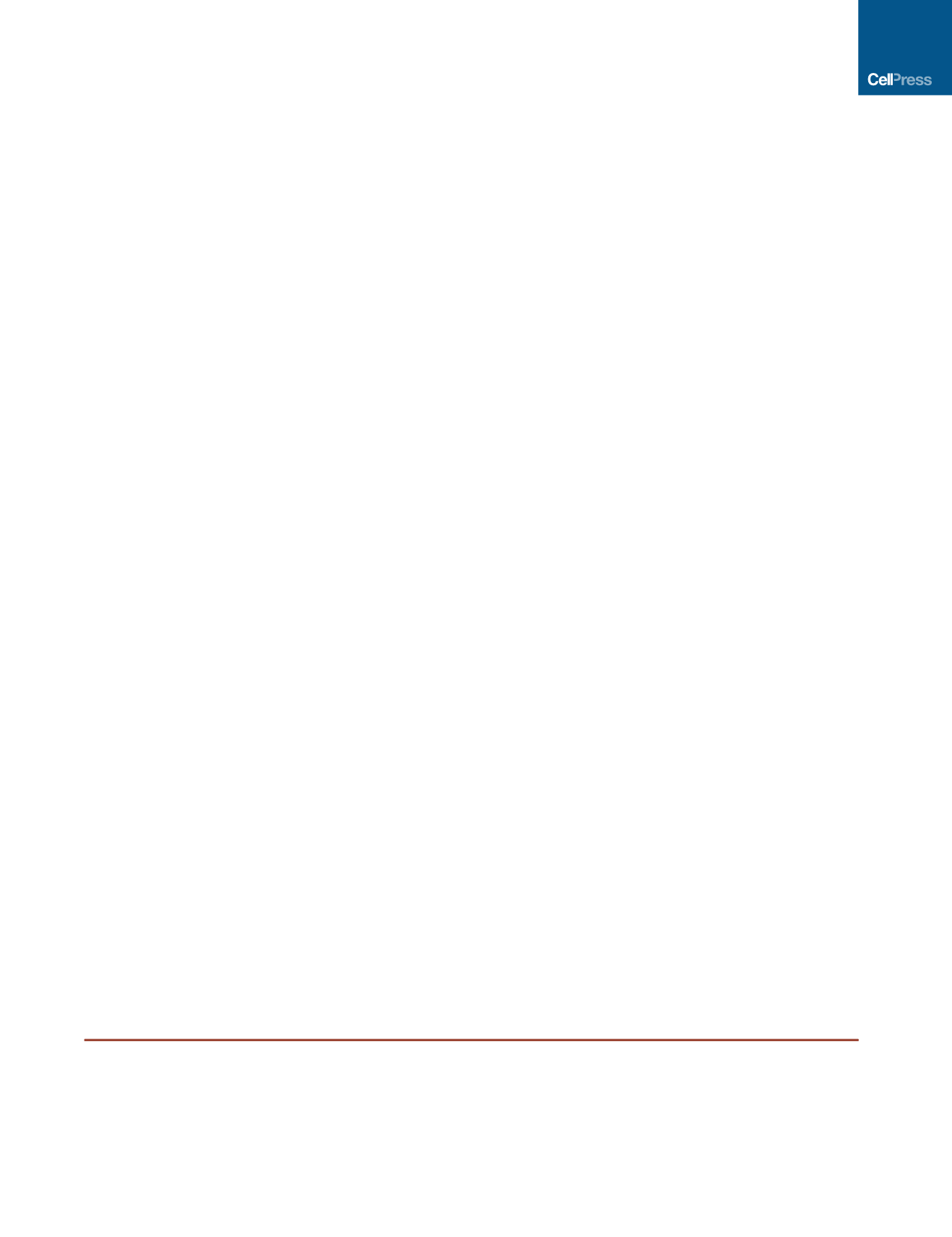
and 6I). All clones showed a marked decrease of the CD41a
+
/
CD45 population (Figure 6I). These phenotypes reflect progres-
sion to low-risk MDS driven by
GATA2
inactivation and to high-
risk MDS driven by the del(7q), suggesting that the del(7q) is a
marker of adverse prognosis in the clinic.
We then set to model disease progression along sequential
stages driven by the stepwise acquisition of genetic lesions
starting from a normal cell. To this end, we first introduced trun-
cating mutations in the
ASXL1
gene and selected two clones
with monoallelic truncations (Figures 6J and S7D).
ASXL1
C-ter-
minal truncation is an early event in myeloid malignancies and
one of the most common mutations in individuals with CHIP
(Link andWalter, 2016; Steensma et al., 2015). We subsequently,
in a second step, deleted one copy of chr7q in one of the
ASXL1
-
engineered clones (Figures 6J and S7E–S7G). This set of clones
recapitulated stepwise progression from normal to preleukemia
(
ASXL1
mutation) to high-risk MDS (
ASXL1
mutation + del7q),
assessed by CD45 and CD90 marker expression, cell viability,
and colony formation (Figures 6K–6N).
These results collectively show that our phenotypic roadmap
can be used to model disease stage transitions across the spec-
trum of myeloid malignancy driven by a variety of genetic lesions
and their combinations.
Modeling Disease-Stage-Specific Effects of
Therapeutic Interventions
5-Azacytidine (5-AzaC) is a hypomethylating agent that is used
as first-line therapy in MDS. 30%–50% of MDS patients show
some response, but there are currently limited biomarkers to
predict the responders (Bejar and Steensma, 2014). Further-
more, the mechanism by which 5-AzaC exerts its therapeutic ef-
fects is not clear. Potential mechanisms may include induction of
differentiation or preferential inhibition of the growth of the MDS
clone. To first test for potential effects of 5-AzaC in inducing dif-
ferentiation, we cultured HPCs derived from the different iPSC
lines in methylcellulose in the presence or absence of 5-AzaC
(Figure 7A). Strikingly, treatment with 5-AzaC resulted in a
marked rescue of BFU-E and CFU-GEMM colonies in low-risk
MDS-iPSCs (Figures 7B, S7H, and S7I). In contrast, it had no ef-
fect in colony growth from normal iPSCs or any other iPSC line
from other disease stages. We then tested for selective effects
in the growth of the MDS clone using a competitive growth
assay. Intriguingly, 5-AzaC had an inhibitory effect in the growth
of high-risk MDS-iPSC-derived HPCs, but not of those derived
from other disease stage iPSCs or normal iPSCs (Figures 7C
and 7D). These results suggest that 5-AzaC may primarily affect
differentiation in earlier stages of the disease, whereas its main
therapeutic action later on might be mediated through selective
inhibition of the MDS clone. DNA methylation analysis of low-risk
MDS-iPSC-derived HPCs (MDS-1.12 line) treated with 5-AzaC
for 3 days revealed striking genome-wide hypomethylation
following 5-AzaC treatment, which included gene promoters,
suggesting that hypomethylation may underlie the rescue of col-
ony formation in these cells (Figures 7E and S7J).
To further test for stage-specific drug responses, we treated
HPCs derived from two MDS/AML lines from patient 4, capturing
a less and a more advanced disease stage, the AML-4.24 line
derived from the dominant clone, and the AML-4.10 line derived
from the KRAS mutated subclone (Figure 1) with rigosertib, a
small-molecule inhibitor of RAS signaling pathways that is
currently in clinical trials for high-risk MDS (Athuluri-Divakar
et al., 2016). As predicted, AML-4.10 HPCs showed marked
sensitivity to rigosertib, whereas AML-4.24 cells were marginally
affected (Figure 7F). These results collectively support the use of
our disease progression model in drug testing.
DISCUSSION
Here, we used an approach integrating cell reprogramming and
cancer genetics to establish iPSC lines representative of distinct
stages during the cellular transformation from normal cells to
AML through an MDS stage. Detailed genetic and clonal charac-
terization of the starting cell population and the derived iPSC
lines allowed us to make additional observations regarding the
degree to which the output of reprogramming is representative
of the clonal composition of the primary cells. Our results show
that the clonal representation of the original cells in the iPSCs
is skewed, often in favor of residual normal cells over cells
of the premalignant or malignant clone (Table S1). They also
show that it is reprogramming per se and not the in vitro stimu-
lation and expansion that accounts for this bias, which seems
to be conferred by some MDS- and AML-associated genetic le-
sions, but not others, while some genetic abnormalities seem to
be incompatible with reprogramming (Figures S1B–S1E). Among
the ones tested here, del(5q) and monosomy 7 could never be
captured in iPSCs, despite cells harboring them comprising
over 80% of the starting cell pool. It might be possible to over-
come this refractoriness by using alternative reprogramming fac-
tor cocktails, which we did not test here. A negative or positive
impact of specific cancer-associated gene mutations on the re-
programming ‘‘fitness’’ of the cells would not be surprising given
well-studied positive and negative effects, respectively, of TP53
inactivation and Fanconi anemia pathway mutations on reprog-
ramming (Papapetrou, 2016). Importantly, despite the skewed
clonal and subclonal representation, we were able to capture
normal and preleukemic cells, as well as malignant clones and
subclones, and thus compile a panel of lines carrying genomes
representative of different disease stages from normal to fully
(C) Schematic of growth competition assay to test the effects of 5-AzaC in cell proliferation relative to normal cells. The cells were mixed 1:1 with the N-2.12 line
stably expressing GFP at day 9 of hematopoietic differentiation in the presence or absence of 5-AzaC and followed for an additional 2 days by flow cytometry.
(D) The relative population size was calculated as the percentage of GFP
-
cells in the treated cells relative to the percentage of GFP cells in the untreated cells at
each time point. iPSC lines from left to right: N-2.12, N-3.10, MDS-1.12, MDS-2.13, and AML-4.24.
(E) Volcano plot showing differences in DNA methylation in four HPC samples independently treated with 5-AzaC derived from the MDS-1.12 line in two inde-
pendent differentiation experiments compared to two untreated controls.
(F) HPCs derived from the AML-4.24 and the AML-4.10 iPSC lines treated with rigosertib. The relative population size was calculated as the number of treated
cells relative to the number of untreated cells at each time point. Mean and SEM from triplicate experiments are shown.
See also Figure S7.
Cell Stem Cell
20
, 315–328, March 2, 2017
325