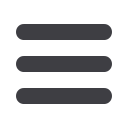
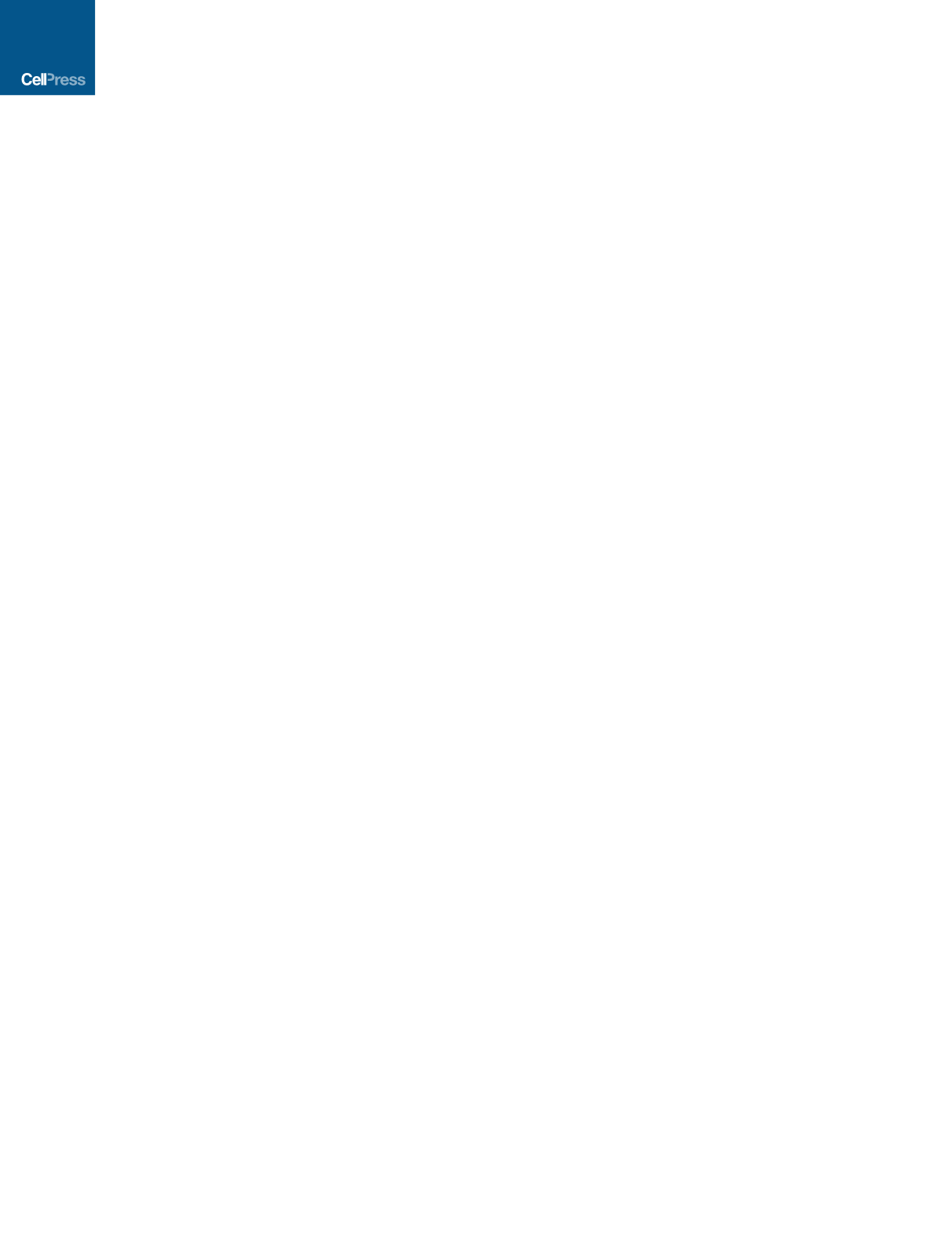
transformed states. Here, we applied this strategy to hemato-
logic malignancies, which are particularly amenable to the
development of a progression model, because they are relatively
genetically simple cancers, and MDS is one of very few well-
recognized pre-neoplastic conditions in humans (Martincorena
and Campbell, 2015). Although the genetic complexity and low
reprogramming efficiency may impose challenges, it is conceiv-
able that similar models can be developed for a variety of other
cancers, including solid tumors (Kim et al., 2013; Kim and Zaret,
2015; Papapetrou, 2016).
The phenotypes we characterized in our model bear direct
relevance to disease phenotypes at the patient level. Morpho-
logic dysplastic changes are a hallmark and a diagnostic
criterion of MDS (Arber et al., 2016). Furthermore, our model
presented a pattern of graded severity from unilineage to
multilineage dysplasia, similar to what is often observed in the
clinic in low-risk versus high-risk MDS cases (Figures 3B and
S5B). The impaired differentiation and reduced clonogenicity
affecting erythroid and multilineage progenitors first is a very
likely correlate of the ineffective hematopoiesis and cytopenias
observed in MDS patients, which predominantly affect the
erythroid lineage, consistent with findings in primary MDS cells
cultured ex vivo (Flores-Figueroa et al., 1999; Sato et al.,
1998). The increased cell death is consistent with findings of
apoptotic markers in primary patient BM, which has led to the
proposition that apoptosis may be another pathophysiologic
mechanism accounting for the cytopenias (Kerbauy and Deeg,
2007). The growth and viability defects of MDS cells are abol-
ished upon transformation to full-blown AML, and this is also
recapitulated in our model. Our findings are also consistent
with previous reports of minimal perturbation of the HSPC
compartment in low-risk MDS but a more significant one in
higher-risk cases (Elias et al., 2014; Will et al., 2012; Woll et al.,
2014). Interestingly, loss of megakaryocyte progenitors is the
earliest event in our progression model, which is intriguing in
view of recent findings on the close relationship between mega-
karyocyte progenitors and HSCs (Notta et al., 2016; Sanjuan-Pla
et al., 2013; Woolthuis and Park, 2016).
Strikingly, hematopoietic cells derived from our MDS/AML-
iPSCs through in vitro differentiation were able to robustly trans-
plant a lethal leukemia when intravenously injected into immu-
nodeficient mice. This is the first demonstration that HSPCs
generated from hPSCs through in vitro differentiation possess
engraftment ability and is an intriguing finding given the general
inability of hematopoiesis derived from human pluripotent stem
cells (hPSCs) to engraft (Vo and Daley, 2015). Deeper investiga-
tion into the transcriptional programs and cellular processes
active in these MDS/AML-iPSC-derived hematopoietic cells
may inform ongoing efforts toward the generation of HSPCs
with long-term engraftment potential from pluripotent or other
cell sources (Vo and Daley, 2015). These cells can also provide
an attractive platform for deconstructing and reconstructing
clonal evolution in AML and for testing drugs in an in vivo setting.
More than a decade ago, it was proposed that myeloid trans-
formation requires two types of events, one that induces prolifer-
ation and one that blocks differentiation, referred to respectively
as class I and II mutations (Gilliland and Griffin, 2002; Gilliland
and Tallman, 2002). The former would typically involve classic
signaling pathways and the latter hematopoietic transcription
factors. It was also suggested that class II without class I muta-
tions might result in MDS. Whereas perturbations of proliferation,
differentiation, and other processes like self-renewal and cell
survival are likely involved in the development of MDS and
MDS/AML, it is now obvious that the picture is much more com-
plex and this model can aid future studies in understanding these
processes at a cellular and molecular level. However, several
limitations need to be noted. MDS is quite heterogeneous genet-
ically and phenotypically, and we only used iPSCs derived from
four patients for this study. Our findings that phenotypes of these
cells cluster with disease stage supports the well-established
observation and long-held idea that diverse genotypes converge
to few phenotypes at the cellular and organismal level in myeloid
malignancies and cancer more generally. Thus, whereas the
derivation of larger collections of MDS and AML iPSC lines in
the future can further refine the phenotypic roadmap we delin-
eate here, our findings can already provide a framework to aid
investigation into disease mechanisms, drug responses, and
the cellular and molecular events driving leukemia progression.
Our results align well with the newly emerging view of myeloid
malignancy as a spectrum of clinical syndromes encompassing
clonal hematopoiesis, MDS, and AML, reflecting disordered he-
matopoietic processes that can often progress from one to
another. However, it is clear that not every patient will necessarily
transition through each of these stages. For example, CHIP can
progress directly to AML without an MDS stage, whereas MDS
and AML can potentially also develop without an antecedent
CHIP phase. It is thus conceivable that different routes to
myeloid transformation exist and that our findings may not apply
to all.
Understanding the cellular events leading to disease stage
transitions can help an enhanced understanding of the process
of myeloid transformation and cellular transformation more
generally and guide drug development targeting specific disease
stages or preventing the progression from one stage to another.
We provided here proof of principle that transitions between
stages (progression or reversal) can be modeled in our system.
Our model offers new opportunities to study HSPC populations
in MDS and AML, which often cannot be easily obtained at suf-
ficient numbers from primary samples or propagated in patient-
derived xenograft models. It also offers the unique opportunity to
study disease mechanisms in pure clonal cells devoid of the con-
founding cellular, genetic, and clonal heterogeneity of primary
patient specimens. Mutation of the second
GATA2
allele upon
progression to MDS has been described in familial cases of
GATA2
mutation, but its role in disease progression has not
been studied before (Collin et al., 2015). Our results suggest
that further loss of function of
GATA2
contributes to progression
(Figures 6G–6I). This is consistent with a fundamental role of
GATA2 in hematopoiesis from studies in mouse models (de Pater
et al., 2013). Our findings, however, also suggest that additional
events are needed for progression to a more aggressive disease
(since
GATA2
knockout [KO] induced rather mild phenotypic
changes; Figures 6H and 6I), which is consistent with the finding
of additional recurrent MDS-associated somatic lesions in the
MDS clone of this patient (Figure 1) and our results showing
more dramatic phenotypic changes driven by engineering a
del(7q) (Figures 6G–6I). While our results using 5-AzaC and rigo-
sertib treatment warrant further investigation, they demonstrate
326
Cell Stem Cell
20
, 315–328, March 2, 2017