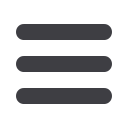
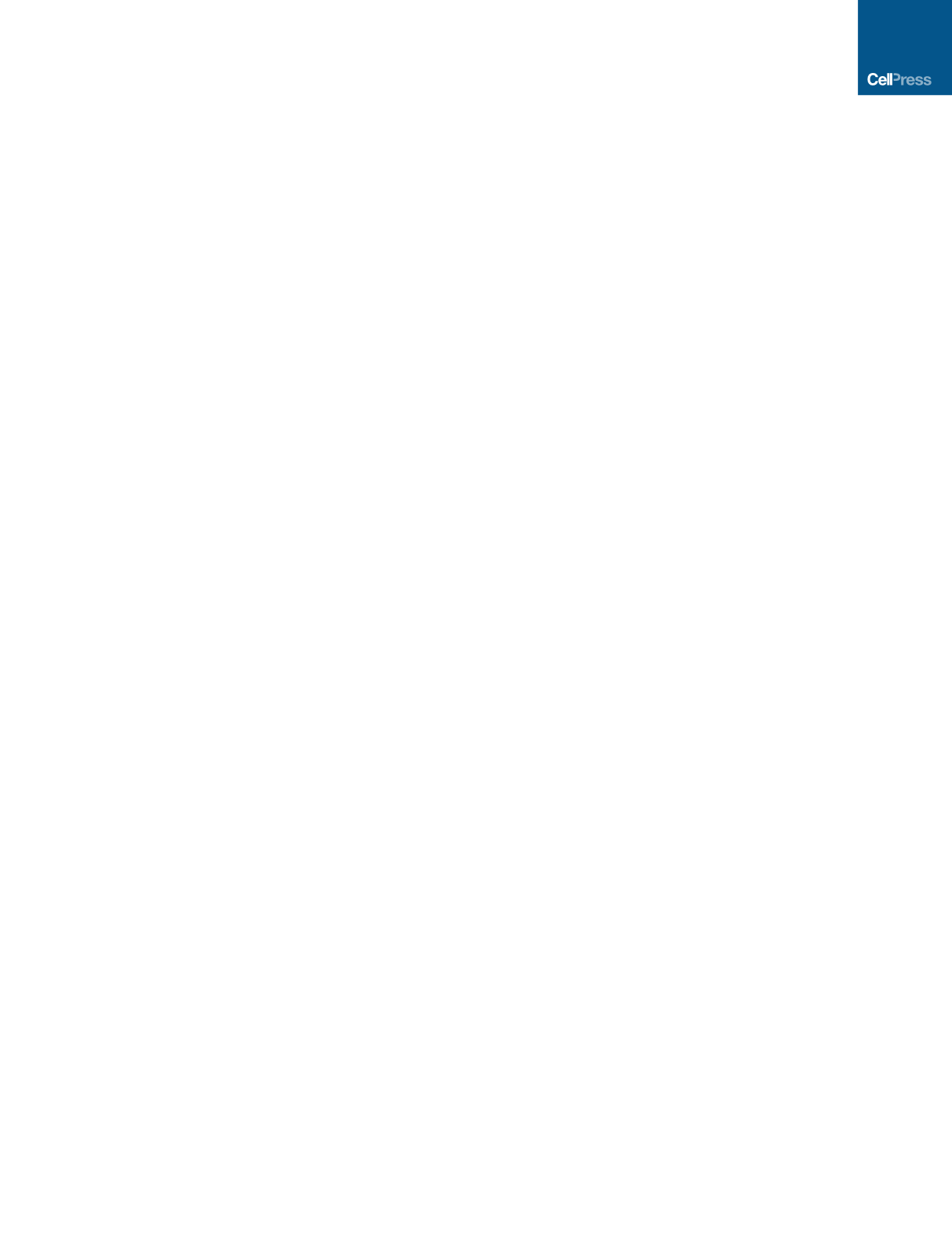
reduced overall efficiency (in contrast to low-risk MDS) (Figures
2C and S3D–S3F). On the other hand, MDS/AML-iPSCs gave
rise to CD45
+
HPCs with efficiencies comparable to those of
normal cells but failed to differentiate further and retained
CD34 expression until day 18 and beyond (Figures 2C and
S3D–S3F). Reciprocally, CD90 expression, normally lost by
day 10–12 of differentiation, was retained by low-risk MDS,
high-risk MDS, and MDS/AML cells in a stage-specific manner
(Figures 2D, S3G, S4A, and S4B).
We found that megakaryocyte progenitors with the CD41a
+
/
CD45 surface phenotype that give rise to CFU-Mk colonies
emerge in these cultures on or before day 8 in normal cells (Fig-
ures 2E–2G, S4B, and S4C). Strikingly, this population was
severely decreased already in preleukemic cells and effectively
abolished in MDS (Figures 2E, S4B, and S4C). While normal
iPSCs gave rise to all types of hematopoietic colonies in methyl-
cellulose cultures (Figures S5A and S5B), iPSCs from all disease
stages exhibited reduced clonogenicity, with erythroid and mul-
tilineage colonies (burst-forming unit-erythrocyte [BFU-E] and
colony-forming unit-granulocyte, erythrocyte, monocyte, mega-
karyocyte [CFU-GEMM]) primarily affected already in preleuke-
mic and, more so, in low-risk MDS cells, while high-risk MDS
generated very few or no colonies (Figures 3A and 3B). MDS/
AML cells gave rise exclusively to myeloid colonies composed
mostly of immature cells (Figures 3A and 3B). Morphologic
assessment of HPCs on day 14 of differentiation and of more
mature cells from methylcellulose cultures revealed dysplastic
changes, which were milder and restricted to the erythroid line-
age in preleukemic and low-risk MDS and more widespread and
affecting all lineages in high-risk MDS cells (Figures 3B and S5C).
Finally, we measured the growth rate and viability of HPCs
derived from the different disease stage iPSCs. Low-risk MDS
showed a mild decrease in growth rate and viability, which was
much more pronounced in high-risk MDS, whereas growth and
viability of MDS/AML cells was completely restored to normal
levels (Figures 3C, 3D, and S5D–S5F).
MDS/AML-Derived Hematopoietic Cells Give Rise to
Serially Transplantable Leukemia
To assess in vivo engraftment potential, we then transplanted
day 8–16 HPCs derived from iPSCs of the various disease stages
into
NOD/SCID/IL-2R
g
/
(NSG) mice (Figure 4A). As expected
from many previous studies, HPCs derived from normal iPSCs
showed no detectable engraftment (Vo and Daley, 2015) (Figures
4B and 4C). Similarly, HPCs from MDS iPSCs (both low-risk
and high-risk) did not exhibit engraftment potential (Figures 4B
and 4C). In contrast, MDS/AML-HPCs showed high levels (up
to 80%) of human engraftment in multiple animals (Figures 4B
and 4C). The transplantable cells showed features of myeloid
leukemia, including a predominantly myeloid immunophenotype
and infiltration of the bone marrow and spleen by immature hu-
man CD45
+
cells with blast-like morphology, also found in the
peripheral blood, which could be transplanted into secondary
recipients (Figures 4D–4H). The latter readily succumbed to an
AML-like disease within 3 weeks of transplantation.
In summary, our phenotypic analyses show that iPSCs derived
from distinct disease stages across the myeloid malignancy
spectrum capture hematopoietic phenotypes of graded severity
and/or stage specificity that together delineate a phenotypic
roadmap to myeloid transformation, ultimately leading to a
fulminant serially transplantable myeloid leukemia (Figure 4I;
Table S3).
Transcriptomes of Disease-Stage-Specific iPSC-
Derived HPCs Recapitulate Features of Disease
Progression
We performed RNA sequencing (RNA-seq) of sorted CD34
+
cells
from three normal lines (two iPSC lines and the H1 hESC line) and
two low-risk MDS, three high-risk MDS, and three MDS/AML
iPSC lines (Figures 5 and S6A). By examining the gene expres-
sion profile among the different disease stages, we found a clear
clustering of samples according to disease status by principal-
component analysis, with the first principal component sepa-
rating normal from MDS and the second separating the AML
from the MDS samples (Figure 5A). Differential expression ana-
lyses identified 472 upregulated and 329 downregulated, 868
upregulated and 284 downregulated, and 760 upregulated
and 439 downregulated genes among AML versus normal,
high-risk MDS versus normal, and low-risk MDS versus normal,
respectively (log2FC > 3 or log2FC < 3 and adjusted P value <
0.05; Figure S6B). Hierarchical clustering of all lines based on
these differentially expressed genes recapitulated progression
from normal to MDS/AML (Figure 5B). Based on analysis of
Gene Ontology (GO) categories, genes involved in positive regu-
lation of apoptosis and negative regulation of cell proliferation
became upregulated at the transition from normal to low-risk
MDS and subsequently downregulated upon transformation
to MDS/AML, in agreement with our phenotypic analyses (Fig-
ure 5C). Similarly, negative regulation of differentiation was a
category upregulated early on. By gene set enrichment analysis
(GSEA), we identified significantly enriched disease-specific and
shared functional pathways in the iPSC-derived HPCs repre-
senting the three different disease stages (Figure S6C; Table
S4). Notably, both the high-risk MDS- and MDS/AML- iPSC-
derived HPCs were significantly enriched for the high-risk MDS
deletion 7q gene set, consistent with both their respective dis-
ease state and their specific genetic makeup (Figure 5D). Addi-
tionally, the MDS/AML-iPSC-HPCs showed specific enrichment
for a gene set found in a subset of human AML patients that had a
worse clinical prognosis and contained chromosome 7 abnor-
malities (Valk et al., 2004) (Figure 5E). Overall, these data suggest
that gene expression programs found in HPCs derived from our
iPSC panel recapitulate disease progression and capture gene
expression signatures derived from primary samples from pa-
tients with myeloid malignancies.
Modeling Disease Stage Transitions
We next asked if this model and the phenotypes characterized
therein could guide modeling transitions between disease
stages, as readouts for disease progression or reversal. We first
analyzed an iPSC line derived from the high-risk MDS line MDS-
2.13 after spontaneous correction of the del(7q) (Kotini et al.,
2015) (Figure 6A). Following correction of the del(7q), this line
only harbors a
SRSF2
P95L mutation (and a
PHF6
mutation of
uncertain significance). Since the
SRSF2
P95L mutation is an
early event in MDS and alone not sufficient for the development
of MDS, the corrected line (MDS-2.A3C) would be predicted to
capture a preleukemic stage (Papaemmanuil et al., 2013).
Cell Stem Cell
20
, 315–328, March 2, 2017
319