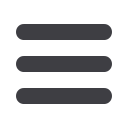
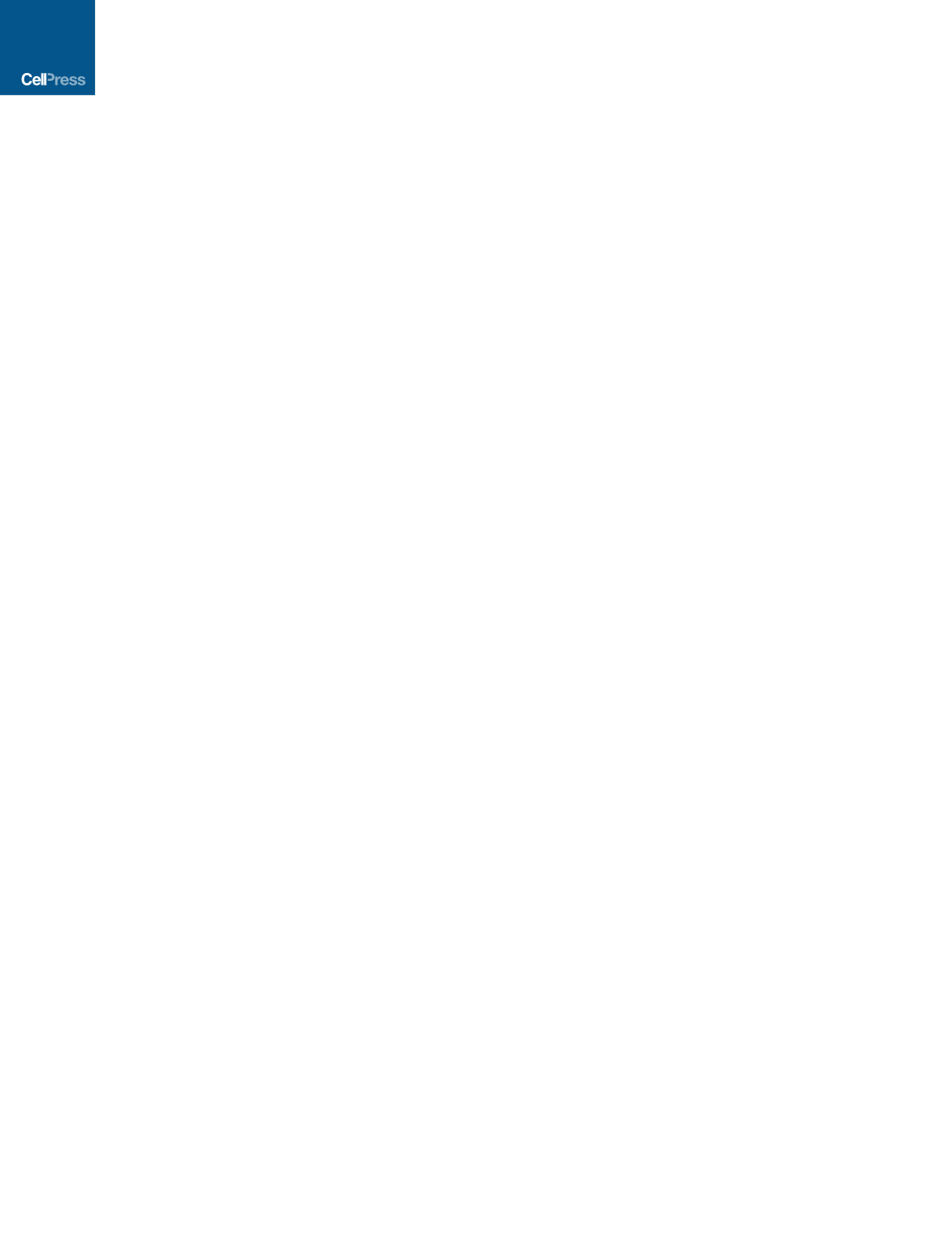
enabled by the identification of tens of recurrent genemutations in
MDS and AML has provided important insights into the nature and
clonality status of myeloid disorders. First, it is now clear that
clonal hematopoiesis is invariably established at the outset of
MDS, and thus MDS is a preleukemic condition not fundamentally
very different from AML (Papaemmanuil et al., 2013; Walter et al.,
2012, 2013). Second, clonal hematopoiesis (termed clonal hema-
topoiesis of indeterminate potential [CHIP]) is found in healthy
individuals with an age-dependent frequency and is associated
with an increased risk of developing MDS, MPD, or AML (Geno-
vese et al., 2014; Jaiswal et al., 2014; Steensma et al., 2015; Xie
et al., 2014). This finding, in parallel with recent functional in vitro
and in vivo studies, lends support to the existence of preleukemic
HSCs that are functionally normal and have multilineage potential
but harbor MDS- and AML-relatedmutations that may give thema
clonal advantage (Jan et al., 2012; Shlush et al., 2014). These
recent findings invite revisiting the boundaries among normal,
premalignant, and malignant hematopoiesis and support an
emerging view of myeloid malignancy as a disease spectrum
comprising hematopoietic disorders that extend across a pheno-
typic continuum, ranging from normal hematopoiesis to clonal
hematopoiesis or preleukemia to MDS and MDS/AML (Pandolfi
et al., 2013; Steensma et al., 2015). However, the cellular events
demarcating progression to overt leukemia through a premalig-
nant myelodysplastic phase are not well defined.
Here, we generated patient-derived induced pluripotent stem
cells (iPSCs) representative of a range of disease stages across
the spectrum of myeloid malignancy, including familial predispo-
sition, low-risk MDS, high-risk MDS, and MDS/AML. We charac-
terized the hematopoiesis derived from this panel of iPSC lines
and identified phenotypes of graded severity and/or stage speci-
ficity, which together delineate a phenotypic roadmap of disease
progression, leading to the most dramatic phenotype of a serially
transplantable leukemia. As proof of principle that transitions be-
tween stages (progression or reversal) can be modeled in our sys-
tem, we show that a high-risk MDS-iPSC line can be phenotypi-
cally reverted to a premalignant state by correction of a chr7q
deletion, whereas a preleukemic iPSC line can progress to either
low-risk or high-riskMDS following CRISPR/Cas9-mediated inac-
tivation of the second
GATA2
allele or deletion of chr7q, respec-
tively. We also model the stepwise progression of normal cells
to preleukemia and subsequent MDS through the sequential intro-
duction of genetic lesions associated with earlier (ASXL1 trunca-
tion) and later (chr7q deletion) disease stages. We then use this
model to uncover disease-stage-specific therapeutic effects of
5-AzaC, a drug usedas first-line therapy inMDS andwhosemech-
anism of action remains elusive, and rigosertib, a small-molecule
inhibitor of RAS signaling. Our study provides insights into the
pathophysiologic changes underlying the initiation and progres-
sion of myeloid transformation and a new platform to test genetic
and pharmacologic interventions to reverse this process.
RESULTS
Integrating Cell Reprogramming with Mutational
Analyses Enables the Generation of Disease-Stage-
Specific iPSCs
We derived iPSC lines from four patients (patients 1–4) with low-
risk MDS (refractory anemia [RA] by French-American-British
classification [FAB]), high-risk MDS (refractory anemia with
excess blasts [RAEB] by FAB) and secondary AML (sAML or
MDS/AML, i.e., AML from preexisting MDS) (Figure 1; Table
S1). For reprogramming, we used BM or peripheral blood (PB)
mononuclear cells (BMMCs or PBMCs) (Table S1) and reasoned
that it might be possible, taking advantage of the genetic and
clonal heterogeneity of these cell populations, to derive iPSC
lines from normal cells, cells of the major clone, as well as cells
from minor subclones. We therefore performed a thorough ge-
netic characterization (karyotype, fluorescence in situ hybridiza-
tion [FISH], array comparative genomic hybridization [aCGH],
and gene mutation analysis) to identify all known recurrent
gene mutations and chromosomal abnormalities associated
with myeloid neoplasms in the starting cells and the derivative
iPSCs and used it to determine the provenance of each iPSC
line (Figure 1). Thus, we were able to establish a variety of
iPSC lines, which included: (1) iPSC lines derived from the domi-
nant clone (i.e., harboring only genetic lesions present in the ma-
jority of the starting cells); (2) iPSC lines derived from sub-clones
(i.e., harboring at least one genetic lesion present in a subset of
the starting cells): AML-4.10, harboring a sub-clonal
KRAS
G12D
mutation, and a second line harboring a sub-clonal
NRAS
Q61R
mutation that could only be partially reprogrammed (Table S1);
(3) iPSC lines derived from normal hematopoietic cells (i.e.,
harboring none of the somatic genetic lesions found in the start-
ing cells); and (4) one iPSC line, N-3.10, derived from patient 3,
harboring a germline
GATA2
T357N mutation predisposing to
MDS/AML (Collin et al., 2015; Hahn et al., 2011) (Table S1).
The MDS clone in this patient had acquired an additional somatic
mutation in the other
GATA2
allele (
GATA2
390delK) (Figure S1A),
together with additional mutations and a t(1;7)(q10;p10) translo-
cation, resulting in del(7q), a deletion commonly associated with
germline
GATA2
mutations (Figure 1) (Wlodarski et al., 2016). All
iPSC lines met all criteria of pluripotency for human cells (Fig-
ure S2). Reprogramming MDS and AML hematopoietic cells
from BM or PB thus allows the derivation of iPSC lines capturing
different disease stages, residual normal cells, and cells with
predisposing mutations.
These reprogramming experiments, together with the genetic
characterization of the original cells and derivative iPSCs, al-
lowed us to make several additional observations.
First, detailed genetic analysis can pinpoint iPSC lines that
originate from the same starting cell and are thus not truly
different lines. iPSC lines MDS-3.4 and MDS-3.5 were found
to both harbor the same
MYB
L51fs mutation, which was not
detectable in the starting population, in addition to the somatic
genetic lesions found in the starting MDS cells (t(1;7)(q10;p10),
GATA2
T357N,
GATA2
390delK,
U2AF1
Q157R,
ETV6
S321fs)
(Figure 1). This strongly suggested that these lines originated
from the same cell, which we confirmed by integration site anal-
ysis of the lentiviral vector used for reprogramming (Kotini et al.,
2015). Second, since our experiments entailed the parallel re-
programming of a mixed population of cells together with the
ability to exclude lines that were not clonally independent (Fig-
ure S2D), we had a unique opportunity to directly compare
the reprogramming efficiency of cells harboring malignancy-
associated genetic lesions to that of normal cells of the same
genetic background and determine how specific genetic lesions
associated with myeloid malignancy may affect reprogramming
316
Cell Stem Cell
20
, 315–328, March 2, 2017