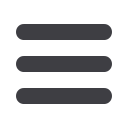
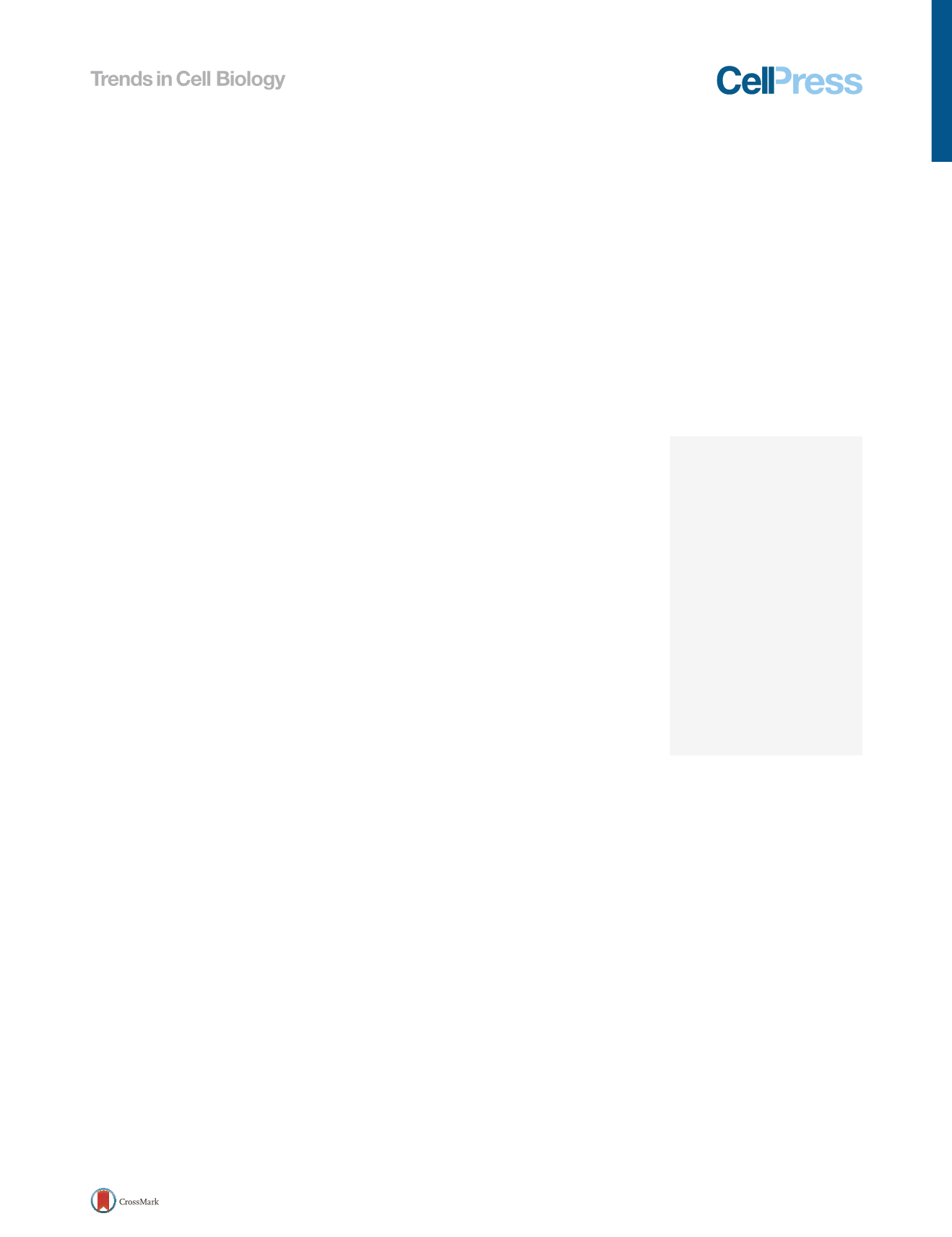
Special Issue: Future of Cell Biology
Review
Applications of CRISPR
Genome Engineering in Cell
Biology
Fangyuan Wang
1
and Lei S. Qi
2,3,4,
*
Recent advances in genome engineering are starting a revolution in biological
research and translational applications. The clustered regularly interspaced
short palindromic repeats (CRISPR)-associated RNA-guided endonuclease
CRISPR associated protein 9 (Cas9) and its variants enable diverse manipu-
lations of genome function. In this review, we describe the development of Cas9
tools for a variety of applications in cell biology research, including the study of
functional genomics, the creation of transgenic animal models, and genomic
imaging. Novel genome engineering methods offer a new avenue to understand
the causality between the genome and phenotype, thus promising a fuller
understanding of cell biology.
From DNA Repair Pathways to CRISPR/Cas9-Mediated Genome Editing
Eukaryotic cells use a sophisticated network of genes and genomic regulatory elements to carry
out functions related to cell growth and death, organelle formation and organization, metabolite
production, and microenvironment sensing. The ability to precisely manipulate the genome is
essential to understanding complex and dynamic cellular processes. Broadly speaking, genome
engineering de
fi
nes methodological approaches to alter genomic DNA sequence (gene editing),
modify epigenetic marks (epigenetic editing), modulate functional output (transcriptional regula-
tion), and reorganize chromosomal structure (structural manipulation) (Figure 1). These goals
require a toolkit of designer molecules that can be conveniently constructed and delivered into
cells to perform one of the above functions.
Naturally occurring systems and pathways have provided a rich resource for tool building. The
discovery of the homology-directed repair (HDR) pathway inspired a method to modify the DNA
sequence at a precise genomic locus in a targeted manner. Using the HDR pathway, a designed
DNA template with
fl
anking homologous sequences could be used to precisely recombine at the
target genomic locus [1]. However, this application is usually a highly inef
fi
cient process in
mammalian cells and tissues. By contrast, the presence of a double-stranded DNA break (DSB)
can enhance ef
fi
ciency [2,3]. Furthermore, it has been shown that, in the absence of a DNA
template, eukaryotic cells may generate almost random deletion or insertion indels at the site of a
DSB via the alternative nonhomology end joining (NHEJ) pathway, offering another approach for
targeted gene knockout [4].
Following the developments described above, a major question in the
fi
eld of gene editing was
how to introduce site-speci
fi
c DSBs to initiate the DNA repair process. Molecules that allow
sequence-speci
fi
c DNA binding were of primary interest. These included programmable
Trends
The RNA-guided CRISPR/Cas9 endo-
nuclease and the endonuclease-dead
dCas9 protein are powerful genomic
[4_TD$DIFF]
manipulation tools for gene editing,
transcriptional regulation, and epige-
netic modi
fi
cations.
Both Cas9 and dCas9 enable diverse
types of high-throughput screening of
gene functions in cell lines and
in vivo.
The CRISPR/Cas9 accelerates the
establishment of many useful trans-
genic animal models for biomedical
research.
The CRISPR/Cas9 is repurposed for
genomic imaging and lineage tracing
in living cells and tissues.
1
Sino-U.S. Center of Synthetic
Biology, Shanghai Institute of
Rheumatology, Renji Hospital, School
of Medicine, Shanghai Jiaotong
University, Shanghai, China
2
Department of Bioengineering,
Stanford University, Stanford, CA
94305, USA
3
Department of Chemical and
Systems Biology, Stanford University,
Stanford, CA 94305, USA
4
ChEM-H, Stanford University,
Stanford, CA 94305, USA
*Correspondence:
stanley.qi@stanford.edu(L.S. Qi).
Trends in Cell Biology, November 2016, Vol. 26, No. 11
http://dx.doi.org/10.1016/j.tcb.2016.08.004875
© 2016 Elsevier Ltd. All rights reserved.