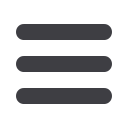
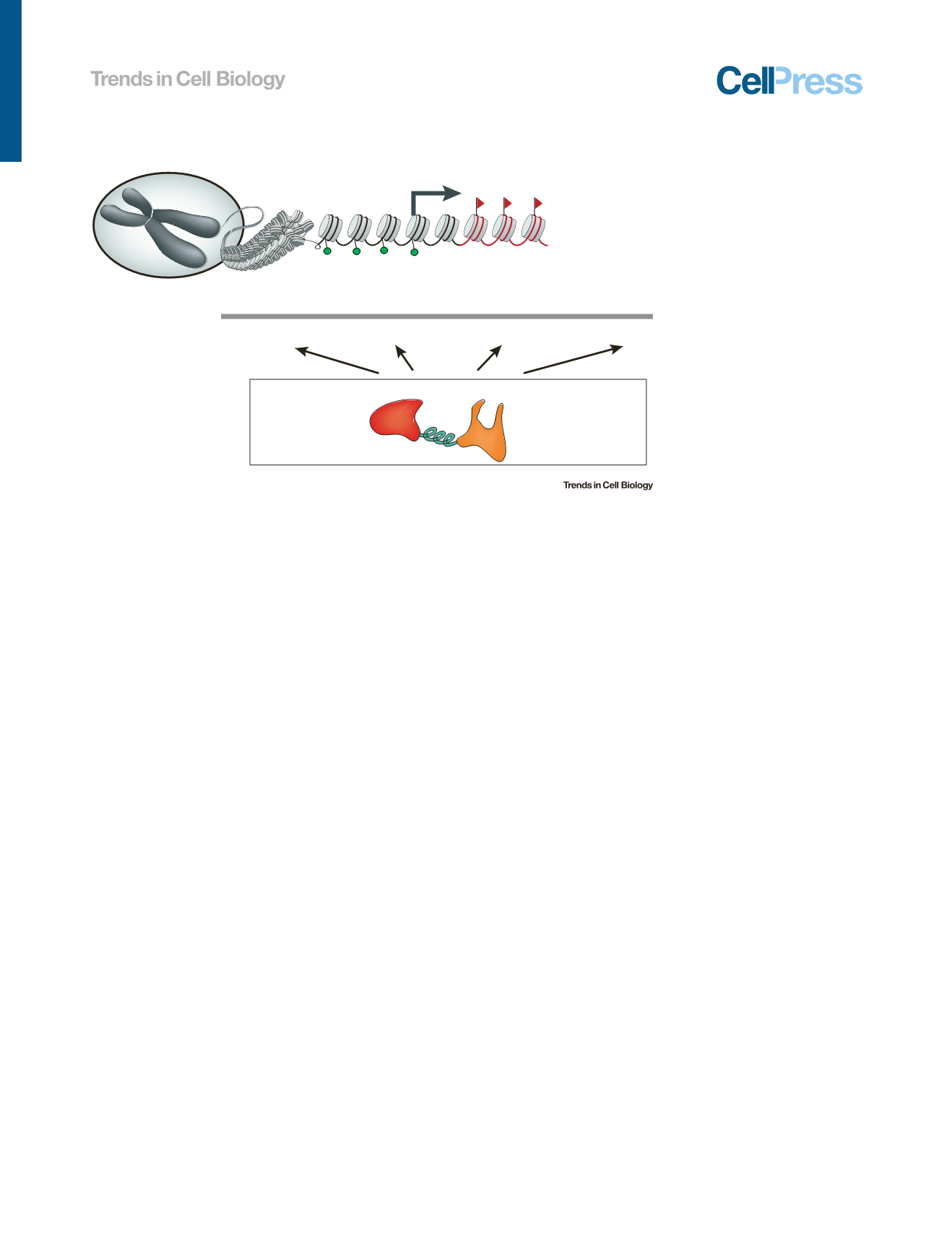
endonucleases engineered from zinc
fi
nger proteins (ZFNs) or transcription activator-like effec-
tors (TALENs) [5,6]. The peptide domains of these proteins could be designed following a simple
set of rules for protein
–
DNA recognition. However, their utility was hindered by an often costly
and tedious construction process and by a context-dependency issue in the protein design
[7,8]. Nevertheless, previous work showed that these programmable DNA-binding proteins
could be coupled to nuclease domains, transcriptional repressors or activators, and epigenetic
modi
fi
ers to enable diverse types of genomic manipulation [9
–
12]. However, it remained to be
understood how to precisely target a speci
fi
c DNA sequence of interest via an even simpler
mechanism, such as Watson-Crick base pairing.
The CRISPR/Cas system performs such a function. Truly a gift from Nature [13,14], the CRISPR/
Cas system was discovered initially in
Escherichia coli
during the 1980s [15], but its function
remained elusive until 2007. Working in the yogurt production bacterium
Streptococcus ther-
mophilus
, earlier work demonstrated that encoding the bacteriophage sequence from the host
CRISPR locus conferred acquired resistance against the same bacteriophage [16]. Later work
showed that CRISPR utilized small CRISPR-associated RNAs (crRNAs) to guide the nuclease
activity of Cas proteins in
E. coli
[17]. Together, these studies uncovered a RNA-guided nuclease
mechanism for the CRISPR system, which also suggested a genetic system with high speci
fi
city
and ef
fi
ciency for DNA binding and cleavage.
The practical use of CRISPR for gene editing began with the elucidation of the mechanism of the
type II CRISPR system [18]. The type II CRISPR from
Streptococcus pyogenes
encodes a RNA-
guided endonuclease protein, Cas9, which was shown to use only two small RNAs (a mature
crRNA and a
trans
-acting tracrRNA) for sequence-speci
fi
c DNA cleavage [18
–
20]. Furthermore,
a chimeric single guide RNA (sgRNA) fused between crRNA and tracrRNA recapitulated the
structure and function of the tracrRNA
–
crRNA complex, which could ef
fi
ciently direct Cas9 to
induce DSBs
in vitro
[18]. The rules used by Cas9 to search for a DNA target are elegant and
simple, requiring only a 20-nucleotide (nt) sequence on the sgRNA that base pairs with the target
DNA and the presence of a DNA protospacer adjacent motif (PAM) adjacent to the complimen-
tary region [18,21].
Nucleus
EpigeneƟc marks
AGCTGACGTG...
Structural
manipulaƟon
TranscripƟonal
regulaƟon
EpigeneƟc
ediƟng
Gene
ediƟng
Different layers of genome engineering
DNA sequence
Promoter
EpigeneƟc marks
Chromosomal loops
or domains
Chromosome
EnzymaƟc domain:
nuclease, transcripƟon
factor, epigeneƟc factor, etc.
DNA-binding domain:
protein or RNA guided
Linker
A toolkit for genome engineering
Figure 1. A Schematic View of the Diverse Goals of Genome Engineering.
Genome engineering de
fi
nes
methodological approaches to alter the DNA sequence (gene editing), modify the epigenetic marks (epigenetic editing),
modulate the functional output (transcriptional regulation), and reorganize the chromosomal structure (structural
manipulation).
876
Trends in Cell Biology, November 2016, Vol. 26, No. 11