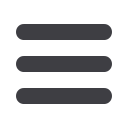
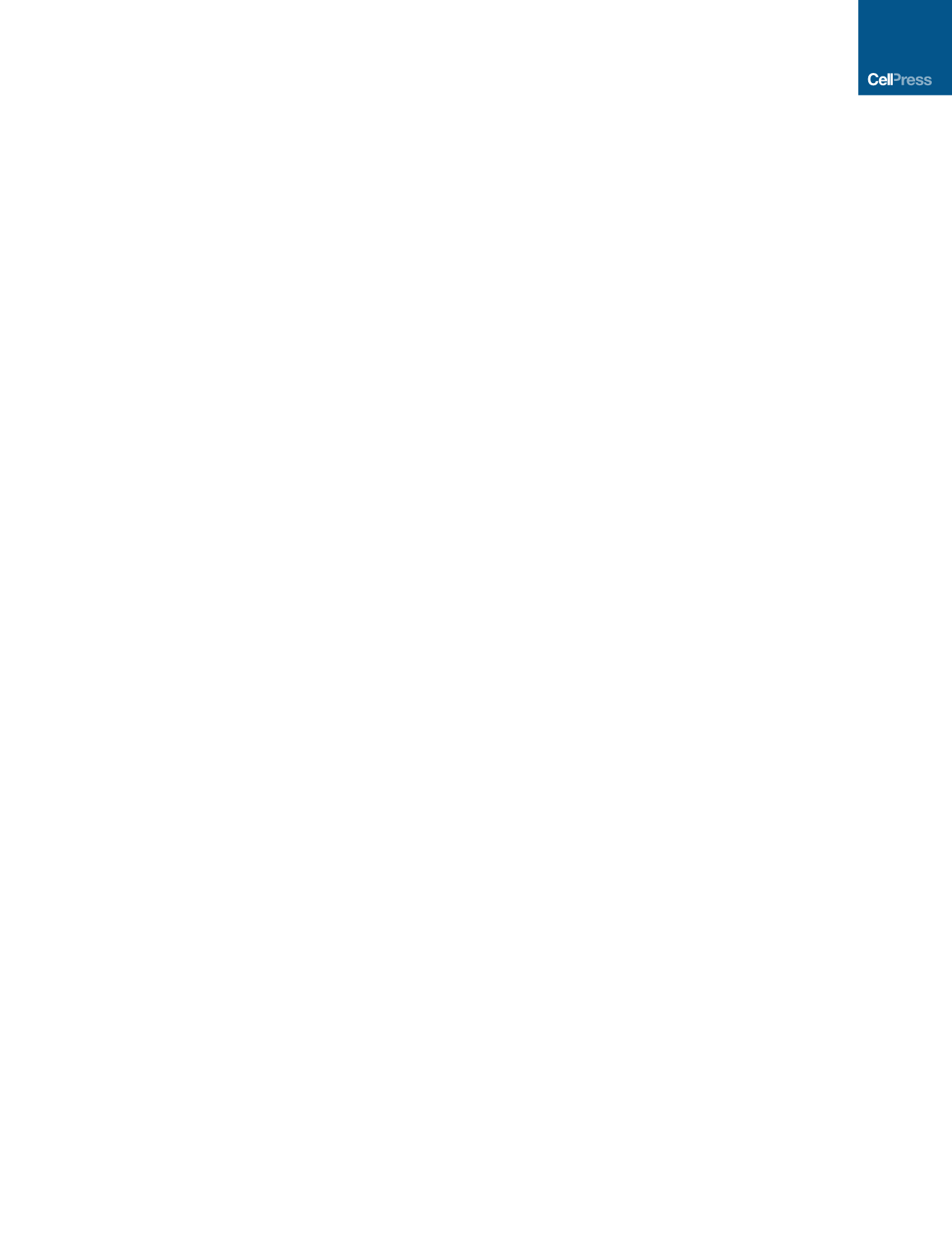
multiplex activation of the neurogenic factors
Brn2
,
Ascl1
, and
Myt1l
(BAM factors). We hypothesized that targeted activation
of the endogenous genes in PMEFs, as opposed to the forced
overexpression of the corresponding transgenes, could more
directly access the endogenous loci and rapidly remodel their
epigenetic signatures, thus potentially reflecting a more natural
mechanism of action and serving as an alternate method to
achieve cell lineage conversion.
In PMEFs, the
cis
-repressive chromatin landscape at neuronal
loci may preclude binding of regulatory factors, in turn impeding
transcriptional activation. As a result, expression of the BAM fac-
tors in PMEFs from exogenous vectors likely relies on stochastic
processes for reactivation of the corresponding endogenous
genes. Furthermore, transient delivery of the BAM factors, as
done in our initial experiments (Figures 1, 2, and 3), limits the
time window within which the endogenous networks and posi-
tive feedback loops can be established. We demonstrated that
targeting the endogenous genes directly induced the enrichment
of histone H3 modifications H3K27ac and H3K4me3 at the
Brn2
and
Ascl1
loci at 3 days post-transfection, whereas transgene
overexpression via transfection of plasmids encoding the re-
programming factors did not alter these chromatin marks (Fig-
ures 3 and S4). Additionally, we observed sustained high levels
of expression from the endogenous genes at later stages of re-
programming despite the transient delivery of the gRNA plas-
mids (Figure S3).
In contrast, we found that stable integration and constitutive
expression of the exogenous reprogramming factors via lentiviral
delivery led to the eventual deposition of H3K27ac at their endog-
enous loci with a concomitant improvement in reprogramming
capacity (Figures 4F and 4G). We did not observe a similar
improvement with constitutive expression of
VP64
dCas9
VP64
and
gRNAs, which is possibly attributable to the lower levels of overall
expression of the neuronal transcription factors achieved by
transactivation of the endogenous genes compared to ectopic
overexpression. Consequently, the direct activation of the endog-
enous genes via
VP64
dCas9
VP64
may be more amenable to tran-
sient delivery approaches that avoid undesired consequences
of vector integration into the genome. Such transient methods,
including the direct delivery of ribonucleoprotein Cas9-gRNA
complexes, may be a more clinically translatable method of
generating reprogrammed cells that are genetically unmodified.
Achieving robust and well-defined reprogrammed cell popula-
tions is still a central challenge. Reprogrammed cells often fail to
acquire completely mature phenotypes and can retain epige-
netic remnants of the native cell type (Kim et al., 2010). Moreover,
a recent study demonstrated that reprogramming efficiency can
be limited by divergence to a competing cell identity (Treutlein
et al., 2016). The molecular mechanisms and practical conse-
quences of these limitations are largely unknown. As the toolkit
of designer transcription factors expands to precisely modify
the epigenome (Hilton et al., 2015; Kearns et al., 2015; Maeder
et al., 2013a; Mendenhall et al., 2013; Thakore et al., 2016), these
tools may be used to prime specific genomic loci in diverse cell
types, promote endogenous transcription factor binding, and
directly correct regions of epigenetic remnants that prove to be
problematic for a given application. This may lead to improved
reprogramming fidelity and extension of the breadth of donor
cells amenable to reprogramming.
EXPERIMENTAL PROCEDURES
Cell Culture, Transfections, and Viral Transductions
PMEFs were maintained in high serum media during transduction and trans-
fection of expression plasmids and subsequently cultured in neurogenic
serum-free medium for the duration of the experiments to promote neuronal
survival and maturation. Lentivirus was produced in HEK293T cells using the
calcium phosphate precipitation method. All transfections were performed us-
ing Lipofectamine 3000 (Invitrogen) in accordance with the manufacturer’s
protocol. All expression plasmids used in this study can be found in Table S2.
Immunofluorescence Staining and qRT-PCR
All sequences for qRT-PCR primers can be found in Table S3. Total RNA
was isolated using the QIAGEN RNeasy and QIAshredder kits, reverse tran-
scribed using the SuperScript VILO Reverse Transcription Kit (Invitrogen),
and analyzed using Perfecta SYBR Green Fastmix (Quanta BioSciences). All
qRT-PCR data are presented as fold change in RNA normalized to
Gapdh
expression. For immunofluorescence staining, cells were fixed with 4% para-
formaldehyde, permeabilized with 0.2% Triton X-100, and incubated with pri-
mary and secondary antibodies.
Electrophysiology
A synapsin-RFP lentiviral reporter was used to identify cells in co-culture with
primary rat astroglia for patch-clamp analysis at indicated time points. Action
potentials and inward and outward currents were recorded in whole-cell config-
uration. Data were analyzed and prepared for publication using pCLAMP and
MATLAB.
Chromatin Immunoprecipitation qPCR
Chromatin was immunoprecipitated using antibodies against H3K27ac and
H3K4me3, and gDNA was purified for qPCR analysis. All sequences for
ChIP-qPCR primers can be found in Table S3. qPCR was performed using
SYBR green Fastmix (Quanta BioSciences), and the data are presented as
fold change gDNA relative to negative control and normalized to a region of
the
Gapdh
locus.
Mouse ENCODE ChIP-Sequencing Datasets
H3K4me3 and H3K27ac ChIP-sequencing data from C57BL/6 E14.5 whole
brain and mouse embryonic fibroblasts (GSE31039) were acquired from the
Mouse ENCODE Consortium generated in Bing Ren’s laboratory at the Ludwig
Institute for Cancer Research.
Statistical Methods
Statistical analysis was done using GraphPad Prism 7. All data were analyzed
with at least three biological replicates and presented as mean ± SEM. See
figure legends for details on specific statistical tests run and p values calcu-
lated for each experiment.
SUPPLEMENTAL INFORMATION
Supplemental Information includes Supplemental Experimental Procedures,
four figures, and three tables and can be found with this article online at
http://dx.doi.org/10.1016/j.stem.2016.07.001.
AUTHOR CONTRIBUTIONS
J.B.B.,A.F.A.,K.W.L.,andC.A.G.designed experiments.J.B.B.,A.F.A.,H.-G.W.,
A.M.D., and H.A.H. performed the experiments. All authors analyzed the data.
J.B.B. and C.A.G. wrote the manuscript with contributions by all authors.
CONFLICTS OF INTEREST
C.A.G. and J.B.B. are inventors on filed patent applications related to this work.
ACKNOWLEDGMENTS
The authors thank Ami Kabadi, Pablo Perez-Pinera, and Syandan Chakraborty
for providing plasmid constructs; Christopher Grigsby for protocols for PMEF
Cell Stem Cell
19
, 406–414, September 1, 2016
413