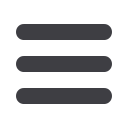
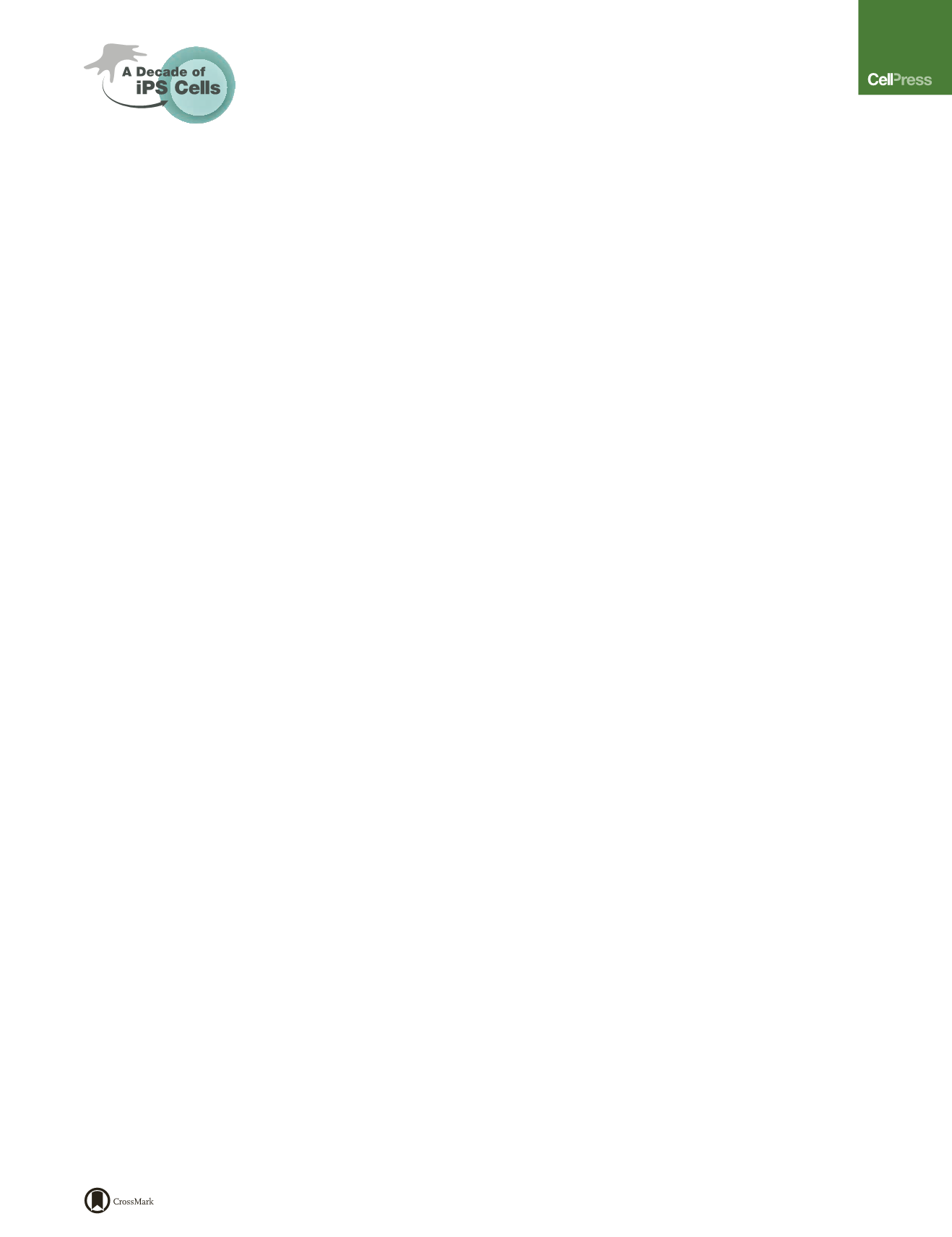
Cell Stem Cell
Review
Induced Pluripotent Stem Cells Meet Genome Editing
Dirk Hockemeyer
1,
* and Rudolf Jaenisch
2
1
Department of Molecular and Cell Biology, University of California, Berkeley, Berkeley, CA 94720, USA
2
The Whitehead Institute for Biomedical Research and Department of Biology, MIT, Cambridge, MA 02142, USA
*Correspondence:
hockemeyer@berkeley.edu http://dx.doi.org/10.1016/j.stem.2016.04.013It is extremely rare for a single experiment to be so impactful and timely that it shapes and forecasts the ex-
periments of the next decade. Here, we review how two such experiments—the generation of human induced
pluripotent stem cells (iPSCs) and the development of CRISPR/Cas9 technology—have fundamentally re-
shaped our approach to biomedical research, stem cell biology, and human genetics. We will also highlight
the previous knowledge that iPSC and CRISPR/Cas9 technologies were built on as this groundwork demon-
strated the need for solutions and the benefits that these technologies provided and set the stage for their
success.
Reprogramming: ‘‘The Yamanaka Experiment’’
Ten years ago Takahashi and Yamanaka reported on the ‘‘Induc-
tion of Pluripotent Stem Cells from Mouse Embryonic and Adult
Fibroblast Cultures by Defined Factors’’ (Takahashi and Yama-
naka, 2006). The hypothesis of this work was daring and stated
that a small set of transcription factors, when ectopically ex-
pressed in a somatic cell, can reprogram it back into a pluripo-
tent state. Retrospectively, the simplicity of the experiments
that Yamanaka and colleagues used to test this hypothesis
was beautiful: take a set of 24 candidate genes, selected mostly
for their high and specific expression in pluripotent cells, and
simultaneously express them in differentiated cells using inte-
grating retroviruses. Identify cells that induced pluripotency via
a selectable marker gene that is not expressed in somatic cells,
but is preferentially activated in pluripotent cells. Next, narrow
down the cocktail of genes to the minimal set of reprogramming
factors (Klf4, Sox2, Oct4, and Myc, a.k.a. KSOM) by the process
of elimination. Lastly, demonstrate that the resulting induced
pluripotent cells have all the key features of their embryonic
stem cell (ECS) counterparts, such as a stem cell-like expression
profile, the ability to give rise to differentiated cells in teratoma
formation assays, and their contribution to tissues in chimeric
mice after blastocyst injections (Takahashi and Yamanaka,
2006).
These experiments had an immediate impact. They came at
a time when the potential of pluripotent stem cells (PSCs) in
research applications and regenerative medicine had widely
been appreciated (Rideout et al., 2002) (Figure 1), but technical
and ethical limitations presented a challenge that severely
impeded major progress toward realizing their full potential. De-
cades before the study by Yamanaka, John Gurdon (Gurdon,
1962, 1963) had demonstrated that the epigenetic profile of a
fully differentiated cell can be reprogrammed to a pluripotent
state. From a set of key experiments Gurdon demonstrated
that a nucleus taken from a differentiated frog cell and injected
into an enucleated oocyte can give rise to a fully developed
frog. This experiment illustrated that during differentiation no
essential genetic material is lost and that the epigenetic changes
that drive cellular differentiation can be reprogrammed to totipo-
tency. Decades later, the cloning of the sheep ‘‘Dolly’’ also by so-
matic cell nuclear transfer (SCNT) demonstrated that Gurdon’s
finding extended to mammals as well (Campbell et al., 1996).
SCNT and cell fusion experiments gave two additional insights
that set the stage for the Yamanaka experiment. First, they
demonstrated that the cytoplasm of an oocyte or an ESC
contained diffusible transacting factors capable of reprogram-
ming a somatic nucleus (reviewed in Ambrosi and Rasmussen,
2005). Second, successful derivation of mice by SCNT with
nuclei of B cells as a donor, which had undergone VDJ-recombi-
nation, provided genetic evidence that terminally differenti-
ated cells can be reprogrammed (Hochedlinger and Jaenisch,
2002). Though more challenging, SCNT was eventually success-
ful in reprogramming human cells into human ESCs (hESCs) in
2014 (Yamada et al., 2014). While these experiments spoke for
the possibility of cellular reprogramming, they also suggested
highly sophisticated machinery and a complex biological pro-
cess, making the success of the basic Yamanaka experimental
approach even more astounding. Even today, the gradual pace
of transcription-factor-mediated reprogramming remains one
of the most fascinating facets of the Yamanaka experiment:
epigenetic changes after fertilization as well as reprogramming
by SCNT occur within a few hours, while reprogramming by
the Yamanaka experiment requires significantly more time,
generally several days and multiple cell divisions. Yet, both pro-
cesses result in a functionally equivalent cellular pluripotent state
in in vitro cultures that is capable of forming an entirely new
organism.
Around the same time as the first mammalian SCNT efforts,
James Thomson derived the first hESC lines (Thomson et al.,
1998). He used a very similar strategy that had proven successful
for Evans and Martin (Evans and Kaufman, 1981; Martin, 1981),
culturing the inner cell mass outgrowth of explanted blastocysts.
However, it is interesting to note that human and mouse ESC
(mESC) maintenance require distinct signaling networks and
culture conditions. LIF/Stat3 is required for maintaining the un-
differentiated state in mESCs and BMP4 can inhibit the MEK/
ERK differentiation pathway resulting in mESC self-renewal. In
contrast hESCs and hiPSCs do not require hLIF, and mainte-
nance of pluripotency seems to rely mostly on FGF and MEK/
ERK signaling, indicating species-specific requirements for
culturing pluripotent cells. It seems likely that this difference
can be attributed to a difference in the developmental stage
Cell Stem Cell
18
, May 5, 2016
ª
2016 Elsevier Inc.
573