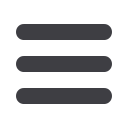
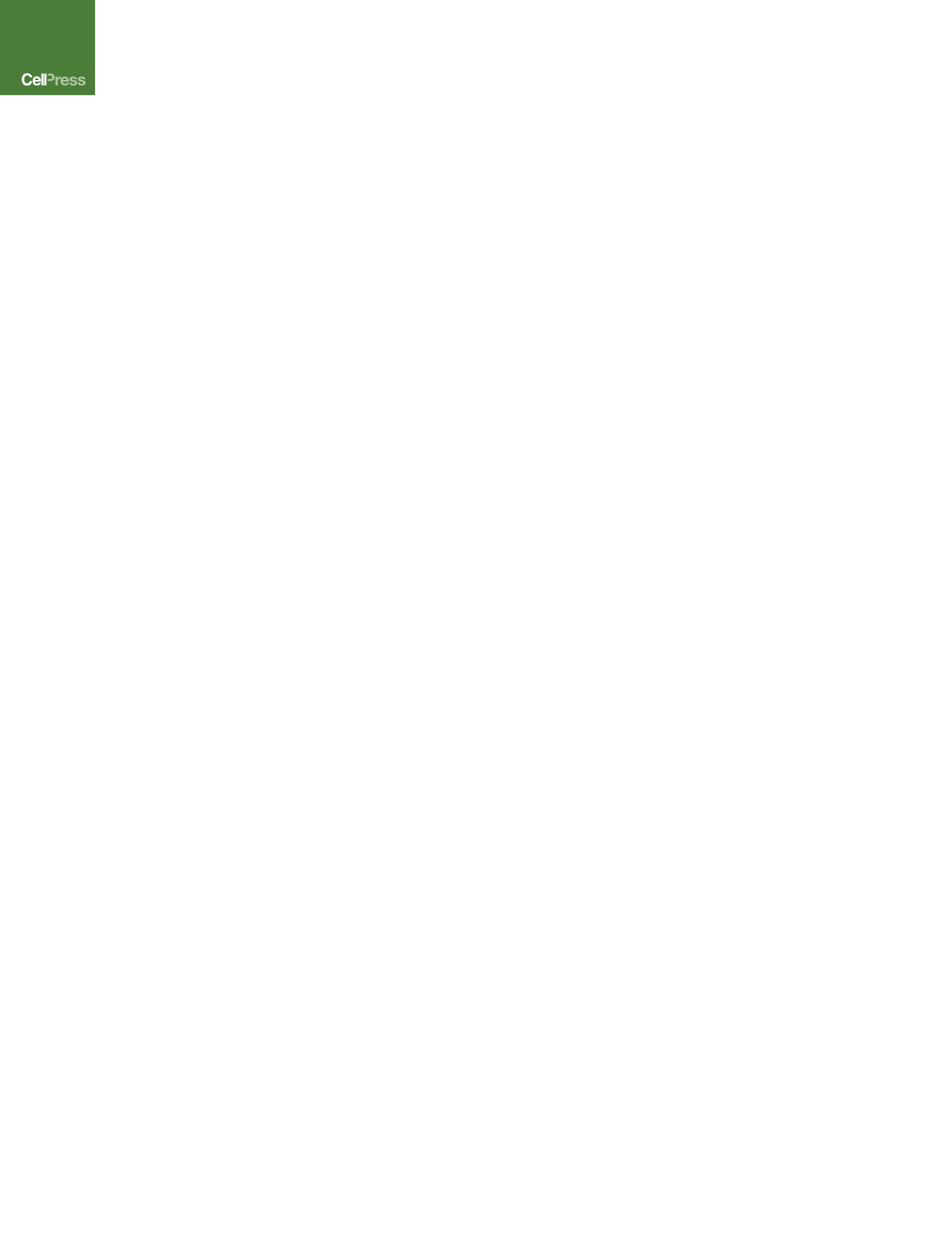
mutations can impact tissue-type-specific cellular behaviors
that are relevant to the particular condition.
For example, this approach has been used successfully to
identify the molecular principles underlying the most frequent
non-coding mutations associated with human cancer (Bojesen
et al., 2013; Fredriksson et al., 2014; Horn et al., 2013; Huang
et al., 2013; Killela et al., 2013). Genetic engineering of these mu-
tations, which occur in the promoter of the catalytic subunit of
human telomerase or TERT, revealed that the mutations result
in the failure of cells to silence TERT transcription upon cellular
differentiation and explains how these mutations function in
tumorigenesis (Chiba et al., 2015).
Gene-correction frequencies in hPSCs are generally much
lower than in tumor cell lines such as K578 or HCT116 cells that
are commonly used for gene editing in cancer cells. A very elegant
approach to overcome this challenge and to increase the effi-
ciency of homology-mediated events in iPSCs was used in exper-
iments that employed zinc finger nucleases to correct mutations in
iPSCs derived frompatients with alpha trypsin deficiency. In these
experiments gene targeting efficiencieswere increasedby the use
of a positive selection marker that allowed the efficient isolation of
the edited clones and that could subsequently be removed
without leaving residual genetic material using piggyBac transpo-
sition. This editing strategy allowed the generation of bi-allelic
editing events in patient-derived iPSCs to restore alpha trypsin
enzymatic function in disease-relevant iPSC-derived hepatocytes
in vitro and after xenotransplantation (Yusa et al., 2011).
A similar approach to overcome the challenges associated
with the low frequency of gene-correction events in hPSCs was
used to correct point mutations in the beta-globin gene of iPSCs
derived from patients with sickle cell disease (Zou et al., 2011). In
this case a LoxP-site flanked selection cassette was used to in-
crease the genome editing efficiency initially, but was then subse-
quently removed using Cre-recombinase. This approach results
in a single residual LoxP site in an intron of the beta-globin
gene. Similarly, two independent studies demonstrated that the
SSN can be used to directly correct
b
-thalassemia mutations in
patient-derived iPSCs and restore hematopoietic differentiation
(Ma et al., 2013; Xie et al., 2014).
Alternative strategies for increasing editing efficiencies include
methods to more efficiently detect and subclone cells that have
undergone rare editing events (Miyaoka et al., 2014) as well as
to enhance deliverymethods for the nuclease and donor template
(Lin et al., 2014). An orthogonal approach to simplify the genera-
tion of isogeneic hPSC lines was the derivation of an inducible
Cas9-expressing cell line by editing a Cas9 expression cassette
into the AAVS1 locus. In this system Cas9 expression can be
induced by doxycycline so that efficient editing afterward only re-
quires the expression or delivery of the sgRNA (Gonza´ lez et al.,
2014). This system has been used to generate loss-of-function al-
leles in EZH2 and to demonstrate the effects of haploinsufficiency
for EZH2 in hematopoietic differentiation (Kotini et al., 2015).
Further developments that facilitate the derivation of genome-
engineered iPSC cell lines are protocols that directly combine
genome editing with reprogramming. Howden et al. demon-
strated that human fibroblasts could be simultaneously reprog-
rammed and edited, resulting in edited iPSCs going through
only one single-cell cloning event without the need for drug
selection (Howden et al., 2015).
Further implementation of gene-editing in patient-specific
iPSCs will have a substantial impact on current disease modeling
approaches. An example of the far-reaching effects is illustrated
by editing experiments that inserted an inducible Xist lncRNA
into chromosome 21 of Down syndrome patient-derived iPSCs.
Using this approach Jiang et al. showed that ectopic expression
of Xist was sufficient to transcriptionally suppress the targeted
third copy of chromosome 21 and to reverse the cellular disease
phenotypes in in vitro differentiated cells (Jiang et al., 2013).
Since the implementation of genome editing in hPSCs, several
diseases have been modeled using isogenic cell lines that have
either corrected a disease-relevant mutation in iPSCs or intro-
duced a disease-relevant allele in wild-type hPSCs. For example,
the genetic correction of mutations in Niemann-Pick type C pa-
tient-specific iPSCs to rescue metabolic defects in cholesterol
metabolismand autophagy, which are responsible for the pathol-
ogy, represents just one demonstration of how this approach has
been successfully implemented (Maetzel et al., 2014). Further-
more, genome editing in hPSCs has been used to establish
models for Rett syndrome disrupting MECP2 function in hPSCs
(Li et al., 2013), to generate HIV-resistant variants alleles of the
CCR5 gene into iPSCs (Ye et al., 2014), to repair MYO15A in
iPSCs derived from patients affected by deafness (Chen et al.,
2016), and to derive isogeneic cell pairs of COL7A1-corrected
iPSCs derived from patients with dystrophic epidermolysis bul-
losa (Sebastiano et al., 2014).
In a growing number of cases, such approaches have also
been used to provide new insight into disease pathology. For
example, SSN-mediated correction of disease-causing muta-
tions in LRKK2 that are associated with Parkinson disease (PD)
revealed the transcriptional changes caused by disease-associ-
ated alleles in patient cells (Reinhardt et al., 2013). Likewise,
genome editing of patient-specific iPSCs followed by in vitro
differentiation was also used to generate an isogenic disease
model for cystic fibrosis by correcting disease-relevant muta-
tions in CFTR followed by differentiation into airway epithelium
(Crane et al., 2015; Firth et al., 2015; Suzuki et al., 2016).
The Challenge of Studying Sporadic (Polygenic)
Diseases
The application of iPSC technology for the study of sporadic dis-
eases poses particular challenges because disease-specific
phenotypic changes are expected to be subtle. The genetic basis
of sporadic or idiopathic diseases is thought to be a combination
of multiple low-effect-size risk alleles, mostly in regulatory
regions such as enhancers, which are identified by GWASs
(Gibson, 2011; Merkle and Eggan, 2013). The ‘‘common dis-
ease-common variant hypothesis’’ proposes that multiple risk
variants with small effect size in combination with additional envi-
ronmental factors are the drivers of sporadic diseases. Thus, a
major challenge of using human-derived cells is that risk variants
are not only present in patients but also in unaffected individuals,
albeit with lower frequency. Thus, individual risk variants are not
sufficient to cause disease-associated phenotypes in carrier indi-
viduals or in hiPSCs derived from carriers or patients. While an
iPSC isolated from a patient would harbor all risk variants that
contribute to the disease, any in vitro study to gain mechanistic
insights is complicated by the high system-immanent variability
in differentiation into the disease-relevant cells (Soldner and Jae-
nisch, 2012). Another complicating factor is that the likely effect of
578
Cell Stem Cell
18
, May 5, 2016
Cell Stem Cell
Review