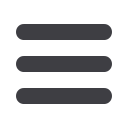
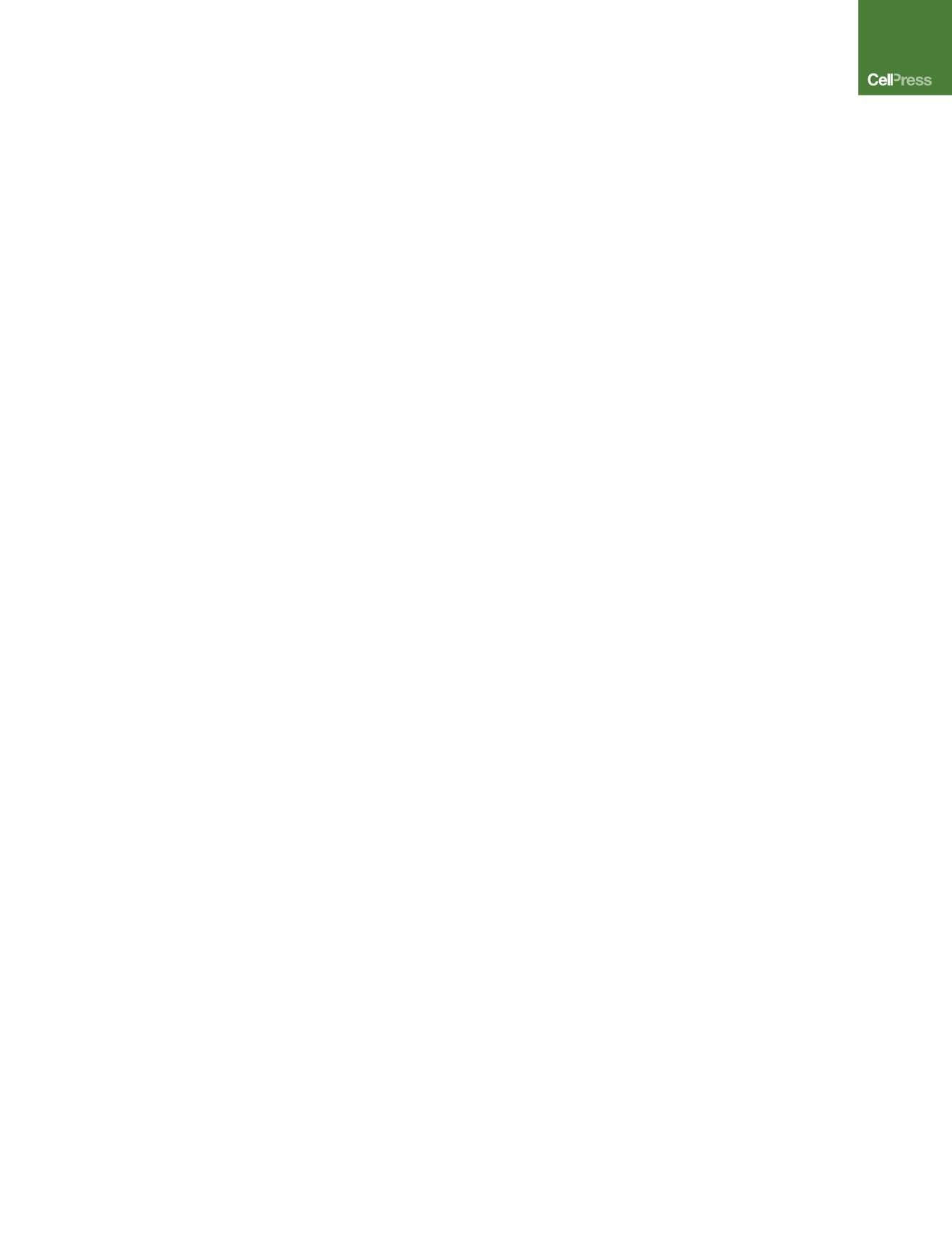
2013a, 2013b; Niu et al., 2014; Wang et al., 2013; Yang et al.,
2013; Yu et al., 2013).
Challenges and Next Steps
Despite the obvious advances that have been made as a result of
iPSC and editing technologies, several challenges remain. A key
limitation remains that human cells prefer to choose the impre-
cise NHEJ pathway to repair a DSB rather than use the more pre-
cise homologous DNA repair pathway using an exogenous repair
template (Chapman et al., 2012). Due to this pathway choice, ed-
iting events often result in NHEJ-mediated insertions and dele-
tions at the DSB rather than the intended homology-mediated
modification. NHEJ-mediated gene disruption can be useful
when the researcher or clinician intends to generate a loss-of-
function event. However, in most clinical treatment settings the
generation of a defined allele with high frequency will be essen-
tial to devise treatment options that require editing to result in
gain of function at endogenous genes. Approaches to shift the
balance away fromNHEJ and toward homology-mediated repair
included inhibiting NHEJ with small molecules or controlling the
timing of CRISPR/Cas9 delivery with respect to the cell-cycle
stage (Chu et al., 2015; Maruyama et al., 2015; Robert et al.,
2015; Yu et al., 2015). These approaches are promising, yet we
are currently far away from testing the efficacy of treatment stra-
tegies that rely on gene repair or gain-of-function approaches
using high-frequency HR repair events of endogenous genes.
Facing this challenge, recent studies used creative ways to
take advantage of NHEJ-meditated genome editing and the
fact that the simultaneous expression of two nucleases can
meditate the excision or inversion of the sequence internal to
the two SSNs (Chiba et al.,2015; Chen et al., 2011; Young
et al., 2016). In the specific case of Duchenne muscular dystro-
phy, Cas9 was employed to excise 725 kb of genomic se-
quences, which removed a premature STOP codon in the
disease-causing DMD gene and thereby restored the reading
frame and partial protein function (Young et al., 2016).
Similarly, Cas9-mediated genome editing in patient-specific
iPSCs was used to genetically correct the disease-causing
chromosomal inversions found in patients with Hemophilia A,
demonstrating that NHEJ-based approaches can be used to
model and correct large-scale genomic alterations underlying
human disease (Park et al., 2015).
Elegant work that also takes advantage of the fact that
genomic sequences between two SSN cuts can reinsert back
into the locus in an inverted manner recently demonstrated
that CTCF sites interact with each other in an orientation-depen-
dent manner (Guo et al., 2015). Using this approach Guo et al.
elucidate the impact of the directionality of CTCF sites in the
mediation of large-scale genome interactions and transcriptional
regulation.
Another challenge of genome editing in human cells is that hu-
man cells have relatively short conversion tracts (Elliott et al.,
1998). This means that even when a DSB is repaired by homol-
ogy-directed repair (HDR) and not the NHEJ machinery, modifi-
cations can only be made with reasonable frequency very close
to one side of the DSB. This presents a major obstacle toward
the introduction of complex genetic changes in hPSCs. The
use of Cpf1, a class 2 CRISPR effector that uses the same basic
principles as Cas9, but cleaves DNA further away from the PAM
sequence and generates a single-stranded overhang, may help
increase the rate of HDR over NHEJ events (Zetsche et al.,
2015). Overcoming this challenge will significantly facilitate the
engineering of human stem cells, as it will allow us to refine the
human genome more efficiently. Eventually this could result in
similar resources that have been used in yeast and mESCs,
such as a comprehensive collection of conditional human
knockout iPSC libraries, with a homozygous iPSC line for each
human gene carrying an exon flanked by LoxP sites.
Rethinking the Ethical Debate
It will be important in the near future to navigate the ethical
debate that arises from the confluence of genome editing
with stem cell technology. This requires a policy framework
that supports scientific progress that is independent of special
interest groups that would bias a rational risk benefit assess-
ment of this technology. The rampant progress that has been
made over the last few years to improve genome editing tech-
nologies and to detect and reduce potential off-targets of SSNs
has already lead to the first clinical trials for HIV, which are trail-
blazing through the necessary regulatory hurdles (Tebas et al.,
2014). Somatic cell editing and editing in hPSCs in vivo and/or
ex vivo coupled with transplantation will progress to become a
standard clinical application. These efforts have to be clearly
distinguished from editing human germ cells or totipotent cells
of the early human embryo. Indeed, the efficiency of altering the
genome of mammals by injecting CRISPR/Cas9 RNA or DNA
into the fertilized egg (Wang et al., 2013) sparked a debate
on whether this technology should be used to modify the
human germline (Sheridan, 2015). While technical challenges
currently limit the potential application of such modifications,
two recent papers describe gene editing of the embryo’s
genome following injection of gRNAs, CRSPR/Cas9 RNA, and
targeting oligos into human zygotes (Kang et al., 2016; Liang
et al., 2015). These studies raise a number of scientific issues
such as off-target rate, mosaicism, and the likely alteration of
the non-targeted wild-type allele when a mutant allele is tar-
geted. More importantly, the technology raises serious ethical
issues: do we want to irreversibly alter the human germline?
Thus, the clinical application of this gene editing technology
for medical purposes raises important ethical issues that will
need to be widely discussed and agreed upon as it would
affect future generations.
ACKNOWLEDGMENTS
We thank the members of the Hockemeyer laboratory for helpful discussion.
D.H. is a New Scholar in Aging of the Ellison Medical Foundation and is
supported by the Glenn Foundation as well as the The Shurl and Kay Curci
Foundations. The work in the Hockemeyer laboratory is supported by
NIH R01 CA196884-01, and in the Jaenisch laboratory, by NIH grants
1R01NS088538-01 and 2R01MH104610-15. R.J. is an advisor to Stemgent
and Fate Therapeutics.
REFERENCES
Ambrosi, D.J., and Rasmussen, T.P. (2005). Reprogramming mediated by
stem cell fusion. J. Cell. Mol. Med.
9
, 320–330.
An, M.C., Zhang, N., Scott, G., Montoro, D., Wittkop, T., Mooney, S., Melov, S.,
and Ellerby, L.M. (2012). Genetic correction of Huntington’s disease pheno-
types in induced pluripotent stem cells. Cell Stem Cell
11
, 253–263.
Cell Stem Cell
18
, May 5, 2016
581
Cell Stem Cell
Review