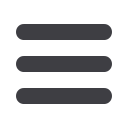
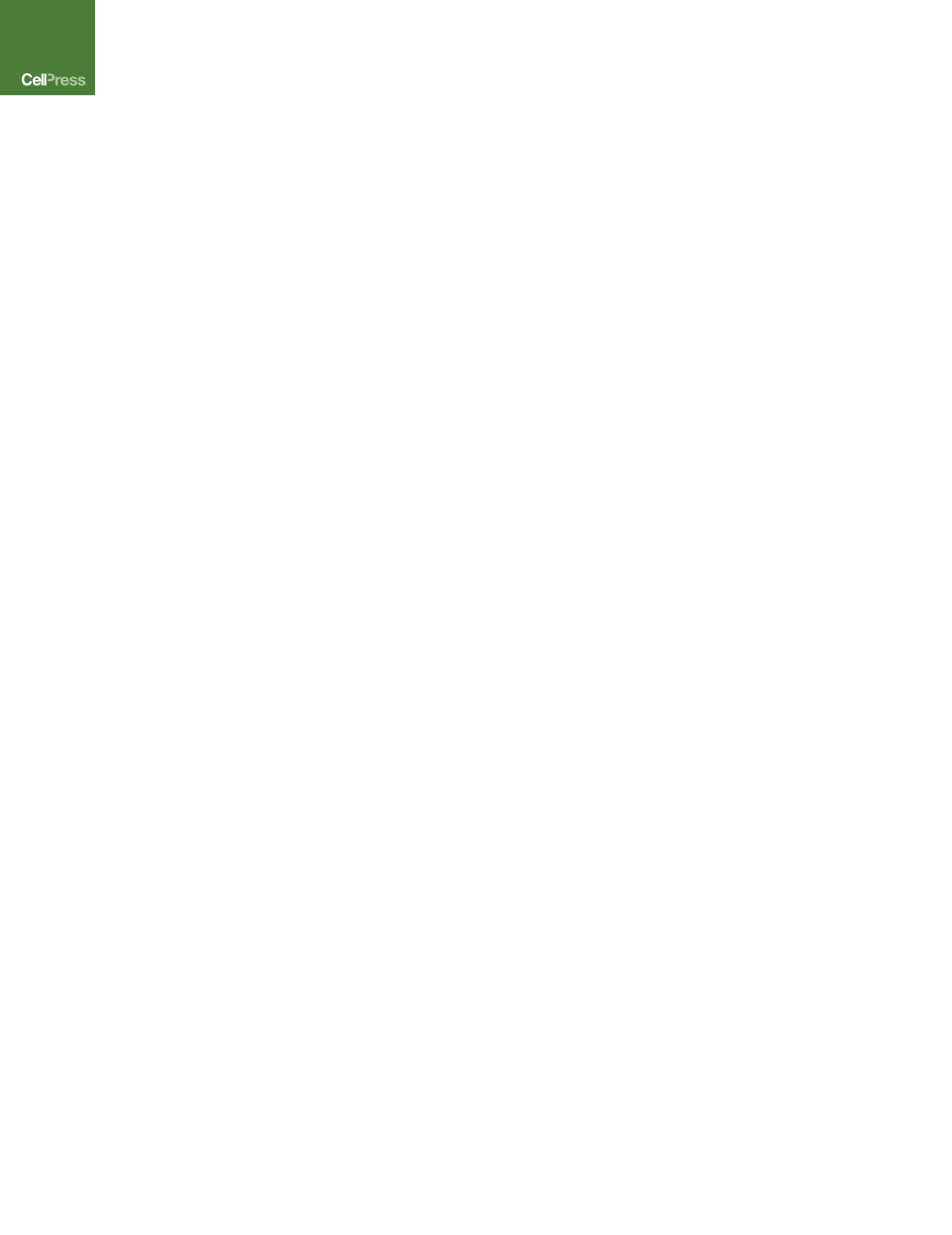
fate decision of differentiating human stem cells (Davis et al.,
2008b; Xue et al., 2009). Overall, however, these approaches
are very time consuming, as they generally require the genera-
tion of large targeting constructs and even then are very inef-
ficient and in many cases not successful. It appears that
cell-intrinsic features such as low homologous recombination
and single-cell survival rates make conventional genome modifi-
cation as described by Capecchi and Smithies for mESCs
(Doetschman et al., 1987; Thomas and Capecchi, 1987) very
inefficient in hPSCs.
Both of these challenges have been overcome: the develop-
ment of the Rho-kinase inhibitor Y-27632 to suppress anoikis
during the disaggregation of hPSC colonies dramatically
increased single-cell survival of hPSCs (Watanabe et al., 2007),
and the low frequency of spontaneous homology-mediated
gene targeting in hPSCs was dramatically increased through
the development of site-specific nucleases (SSNs) as a tool for
their genetic engineering (reviewed in Carroll, 2014; Hsu et al.,
2014; Urnov et al., 2010).
Genome Editing BC (Before CRISPR/Cas9)
The development of SSNs as research tools parallels the devel-
opment of iPSCs: key experiments uncovered the biological
principles and highlight how a generalized platform for genome
editing would advance basic and biomedical research. Repur-
posing of the CRISPR/Cas9 system as an engineered SSN
removed the impediments that limited the full potential of
genome editing by providing this general platform.
Key experiments more than 15 years ago in mammalian cells
demonstrated that a double-strand break (DSB) generated by
an SSN at a defined genomic site can be repaired either by
the endogenous homology-mediated repair machinery using
an exogenous provided repair template or by the error-prone
non-homologous end joining (NHEJ)-DNA repair pathway (Rouet
et al., 1994a, 1994b). The crucial observation made during these
experiments was that a DSB increased the rate of homology-
mediated genomic changes at the break site by several orders
of magnitude compared to conditions in which only an exoge-
nous repair template was provided without the induction of a
DSB. Importantly, this principle of employing a DSB to facilitate
DNA-repair mediated editing of genomes proved to be almost
universal and applies to hPSCs as well as other systems such
as
Caenorhabditis elegans
(Morton et al., 2006; Wood et al.,
2011) and
Drosophila melanogaster
(Beumer et al., 2008; Bibi-
kova et al., 2002, 2003), which are similarly resilient to conven-
tional gene-targeting strategies as hPSCs are.
Already in 2005, Urnov et al. demonstrated that engineered
zinc finger nucleases (ZFNs) can serve as a designer SSN to cor-
rect X-linked SCID disease-relevant mutations in patient-spe-
cific cells (Urnov et al., 2005). It was this study that coined the
term ‘‘genome editing.’’ Ten years later the first clinical trials
based on this ZFN technological platform are underway to
disrupt CCR5 in T cells to treat HIV patients (Tebas et al., 2014).
Based on these pioneering experiments, we and others imple-
mented the use of SSNs such as ZFNs and transcription acti-
vator-like effector nucleases (TALENs) to engineer hPSCs
(DeKelver et al., 2010; Hockemeyer and Jaenisch, 2010; Hocke-
meyer et al., 2009, 2011; Lombardo et al., 2007; Sexton et al.,
2014; Soldner et al., 2011; Zou et al., 2009). These experiments
provided proof of principle for SSN-mediated gene knockouts,
for the insertion of transgenes into expressed and non-ex-
pressed genes to generate cell-type-specific lineage reporters,
for the overexpression of transgenes from genetically defined
loci, and for the insertion or repair of disease-relevant point mu-
tations in hPSCs (Figure 2).
The technical advances that established genetic control in
hPSCs proved to be highly synergistic with the development of
iPSC technology. Genome editing in hPSCs overcame the issue
of enormous genetic background variability inherent in iPSC-
based disease models. Independent proof-of-concept studies
demonstrated that SSNs can be used to repair or introduce dis-
ease-relevant mutations in hPSCs (Soldner et al., 2011; Yusa
et al., 2011). The resulting pairs of PSC lines are isogenic, except
for the disease-relevant mutation. Parallel differentiation of such
isogenic sets of cells into disease-relevant cells and tissues can
be used to directly assess the contribution of a mutation to
cellular pathology (Chung et al., 2013; Ryan et al., 2013; Wang
et al., 2014b; Yusa et al., 2011).
The initial ZFN and TALEN platforms to generate SSNs for
genome editing in stem cells were costly and labor intensive
and their implementation as research tools therefore developed
comparatively slowly. However, extensive work with ZFNs and
TALENs has demonstrated the power of genome editing and
highlighted the impact that a universal, cheaper, and simpler
platform to make SSNs would have.
CRISPR/Cas9: Everyone Can Edit Anything
The need for a simple and unified platform to generate SSNs was
met and resolved, similarly to the need for an easy way to make
iPSCs, through a single experiment: by repurposing the bacte-
rial Clustered regularly interspaced short palindromic repeats
(CRISPR)/CRISPR-associated (Cas) adaptive immune systems
(reviewed in Marraffini, 2015) as an SSN. In 2012 the collabora-
tive work of the Jennifer Doudna and Emmanuelle Charpentier
laboratories demonstrated that in CRISPR type-2 systems a sin-
gle protein, Cas9, can function as a designer SSN by associating
with an engineered single guide RNA (sgRNA) that bears homol-
ogy to a genetic locus of interest (Jinek et al., 2012). In this
process, the sgRNA substitutes the natural Cas9-associated
bacterial RNAs that normally confer target specificity for the bac-
terial pathogen DNA and instead directs Cas9 to introduce a
blunt DSB in any target DNA with complementarity to a 20-nucle-
otide (nt)-long sequence in the sgRNA. Doudna and colleagues
predicted that this simple way of engineering SSNs could be ex-
ploited to streamline genome editing (Jinek et al., 2012). In less
than 4 years this prediction became reality and Cas9-mediated
genome engineering was developed into the platform of choice
to generate SSNs and to genetically modify hPSCs (Chen
et al., 2015; Cong et al., 2013; Fu et al., 2014; Gonza´ lez et al.,
2014; Kleinstiver et al., 2015; Hou et al., 2013; Hsu et al., 2014;
Kleinstiver et al., 2016; Liao and Karnik, 2015; Lin et al., 2014;
Mali et al., 2013; Ran et al., 2015; Slaymaker et al., 2016; Tsai
et al., 2014; Wu et al., 2014b). Some important adaptations
and improvements to increase the ease and scope of Cas9-
mediated genome engineering in hPSCs were the establishment
of CRISPR/CAS-systems from different organisms (Hou et al.,
2013; Zetsche et al., 2015) that respond to different PAM
sequences and the engineering of spCas9 to associate with
576
Cell Stem Cell
18
, May 5, 2016
Cell Stem Cell
Review