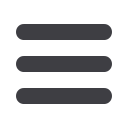
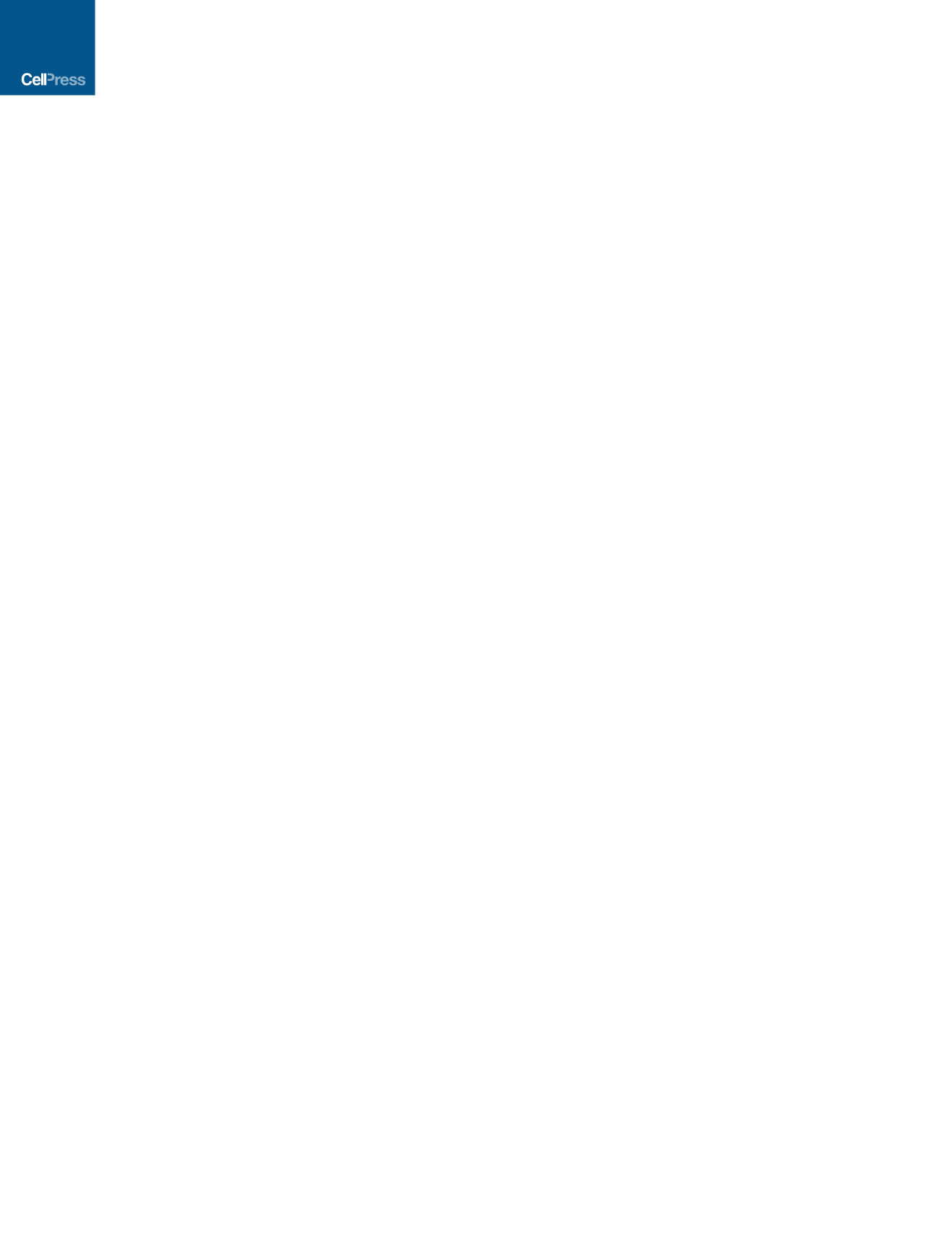
ACKNOWLEDGMENTS
We would like to thank Doug Black, Richard Wang, and Haibin Xi for sugges-
tions and Diana Becerra and Jane Wen for technical assistance. The following
cores were utilized: CDMD Muscle Phenotyping and Imaging Core, HTS and
Cell Repository Core, and Bioinformatics and Genomics Core (supported by
NIAMS-P30 AR057230); the UCLA Broad Stem Cell Research Center (BSCRC)
Flow Cytometry Core Resource, the UCLA Jonsson Comprehensive Cancer
Center (JCCC), and Center for AIDS Research (CFAR) Flow Cytometry Core
Facility (supported by NIH P30 CA016042, 5P30 AI028697); and the UCLA In-
tegrated Molecular Technologies Core (supported by CURE/P30 DK041301),
the UCLA Humanized Mouse Core (supported by the JCCC, BSCRC, CFAR,
and NIH/NIAID AI028697), and the UCLA GenoSeq core. This material is based
upon work supported by the National Science Foundation Graduate Research
Fellowship Program under Grant No. DGE-1144087 (C.S.Y.). Funding was pro-
vided by NIAMS of the NIH (P30 AR057230 to M.J.S., A.D.P., and A.N. and
R01AR064327 to A.D.P.), the Eli and Edythe Broad Center of Regenerative
Medicine and Stem Cell Research at UCLA (M.J.S. and A.D.P.), and a Rose
Hills Foundation Research Award (A.D.P.).
Received: September 9, 2015
Revised: December 18, 2015
Accepted: January 22, 2016
Published: February 11, 2016
REFERENCES
Arechavala-Gomeza, V., Anthony, K., Morgan, J., and Muntoni, F. (2012).
Antisense oligonucleotide-mediated exon skipping for Duchenne muscular
dystrophy: progress and challenges. Curr. Gene Ther.
12
, 152–160.
Be´ roud, C., Tuffery-Giraud, S., Matsuo, M., Hamroun, D., Humbertclaude, V.,
Monnier, N., Moizard, M.P., Voelckel, M.A., Calemard, L.M., Boisseau, P.,
et al. (2007). Multiexon skipping leading to an artificial DMD protein lacking
amino acids from exons 45 through 55 could rescue up to 63% of patients
with Duchenne muscular dystrophy. Hum. Mutat.
28
, 196–202.
Bladen, C.L., Salgado, D., Monges, S., Foncuberta, M.E., Kekou, K., Kosma,
K., Dawkins, H., Lamont, L., Roy, A.J., Chamova, T., et al. (2015). The
TREAT-NMD DMD Global Database: analysis of more than 7,000 Duchenne
muscular dystrophy mutations. Hum. Mutat.
36
, 395–402.
Cacchiarelli, D., Incitti, T., Martone, J., Cesana, M., Cazzella, V., Santini, T.,
Sthandier, O., and Bozzoni, I. (2011). miR-31 modulates dystrophin expres-
sion: new implications for Duchenne muscular dystrophy therapy. EMBO
Rep.
12
, 136–141.
Carsana, A., Frisso, G., Tremolaterra, M.R., Lanzillo, R., Vitale, D.F., Santoro,
L., and Salvatore, F. (2005). Analysis of dystrophin gene deletions indicates
that the hinge III region of the protein correlates with disease severity. Ann.
Hum. Genet.
69
, 253–259.
Cradick, T.J., Qiu, P., Lee, C.M., Fine, E.J., and Bao, G. (2014). COSMID: A
web-based tool for identifying and validating CRISPR/Cas off-target sites.
Mol. Ther. Nucleic Acids
3
, e214.
Dekel-Naftali, M., Aviram-Goldring, A., Litmanovitch, T., Shamash, J., Reznik-
Wolf, H., Laevsky, I., Amit, M., Itskovitz-Eldor, J., Yung, Y., Hourvitz, A., et al.
(2012). Screening of human pluripotent stem cells using CGH and FISH reveals
low-grade mosaic aneuploidy and a recurrent amplification of chromosome
1q. Eur. J. Hum. Genet.
20
, 1248–1255.
Echigoya, Y., Aoki, Y., Miskew, B., Panesar, D., Touznik, A., Nagata, T.,
Tanihata, J., Nakamura, A., Nagaraju, K., and Yokota, T. (2015). Long-term ef-
ficacy of systemic multiexon skipping targeting dystrophin exons 45-55 with a
cocktail of vivo-morpholinos in mdx52 mice. Mol. Ther. Nucleic Acids
4
, e225.
Guan, X., Mack, D.L., Moreno, C.M., Strande, J.L., Mathieu, J., Shi, Y.,
Markert, C.D., Wang, Z., Liu, G., Lawlor, M.W., et al. (2014). Dystrophin-defi-
cient cardiomyocytes derived from human urine: new biologic reagents for
drug discovery. Stem Cell Res. (Amst.)
12
, 467–480.
Harper, S.Q., Hauser, M.A., DelloRusso, C., Duan, D., Crawford, R.W., Phelps,
S.F., Harper, H.A., Robinson, A.S., Engelhardt, J.F., Brooks, S.V., and
Chamberlain, J.S. (2002). Modular flexibility of dystrophin: implications for
gene therapy of Duchenne muscular dystrophy. Nat. Med.
8
, 253–261.
Li, H.L., Fujimoto, N., Sasakawa, N., Shirai, S., Ohkame, T., Sakuma, T.,
Tanaka, M., Amano, N., Watanabe, A., Sakurai, H., et al. (2015). Precise
correction of the dystrophin gene in duchenne muscular dystrophy patient
induced pluripotent stem cells by TALEN and CRISPR-Cas9. Stem Cell
Reports
4
, 143–154.
Long, C., McAnally, J.R., Shelton, J.M., Mireault, A.A., Bassel-Duby, R., and
Olson, E.N. (2014). Prevention of muscular dystrophy in mice by CRISPR/
Cas9-mediated editing of germline DNA. Science
345
, 1184–1188.
Long, C., Amoasii, L., Mireault, A.A., McAnally, J.R., Li, H., Sanchez-Ortiz, E.,
Bhattacharyya, S., Shelton, J.M., Bassel-Duby, R., and Olson, E.N. (2016).
Postnatal genome editing partially restores dystrophin expression in a mouse
model of muscular dystrophy. Science
351
, 400–403.
Menke, A., and Jockusch, H. (1995). Extent of shock-induced membrane
leakage in human and mouse myotubes depends on dystrophin. J. Cell Sci.
108
, 727–733.
Nakamura, A., Yoshida, K., Fukushima, K., Ueda, H., Urasawa, N., Koyama, J.,
Yazaki, Y., Yazaki, M., Sakai, T., Haruta, S., et al. (2008). Follow-up of three pa-
tients with a large in-frame deletion of exons 45-55 in the Duchenne muscular
dystrophy (DMD) gene. J. Clin. Neurosci.
15
, 757–763.
Nelson, C.E., Hakim, C.H., Ousterout, D.G., Thakore, P.I., Moreb, E.A., Rivera,
R.M.C., Madhavan, S., Pan, X., Ran, F.A., Yan, W.X., et al. (2016). In vivo
genome editing improves muscle function in a mouse model of Duchenne
muscular dystrophy. Science
351
, 403–407.
Ousterout, D.G., Kabadi, A.M., Thakore, P.I., Majoros, W.H., Reddy, T.E., and
Gersbach, C.A. (2015). Multiplex CRISPR/Cas9-based genome editing for
correction of dystrophin mutations that cause Duchenne muscular dystrophy.
Nat. Commun.
6
, 6244.
Partridge, T. (2002). Myoblast transplantation. Neuromuscul. Disord.
12
(
Suppl
1
), S3–S6.
Pearce, J.M.S., Pennington, R.J.T., and Walton, J.N. (1964). Serum enzyme
studies in muscle disease: Part III Serum creatine kinase activity in relatives
of patients with the Duchenne type of muscular dystrophy. J. Neurol.
Neurosurg. Psychiatry
27
, 181–185.
Tabebordbar, M., Zhu, K., Cheng, J.K.W., Chew, W.L., Widrick, J.J., Yan,
W.X., Maesner, C., Wu, E.Y., Xiao, R., Ran, F.A., et al. (2016). In vivo gene edit-
ing in dystrophic mouse muscle and muscle stem cells. Science
351
, 407–411.
Taglia, A., Petillo, R., D’Ambrosio, P., Picillo, E., Torella, A., Orsini, C., Ergoli,
M., Scutifero, M., Passamano, L., Palladino, A., et al. (2015). Clinical features
of patients with dystrophinopathy sharing the 45-55 exon deletion of DMD
gene. Acta Myol.
34
, 9–13.
Wojtal, D., Kemaladewi, D.U., Malam, Z., Abdullah, S., Wong, T.W.Y., Hyatt,
E., Baghestani, Z., Pereira, S., Stavropoulos, J., Mouly, V., et al. (2016).
Spell Checking Nature: Versatility of CRISPR/Cas9 for Developing
Treatments for Inherited Disorders. Am. J. Hum. Genet.
98
, 90–101.
Xu, L., Park, K.H., Zhao, L., Xu, J., El Refaey, M., Gao, Y., Zhu, H., Ma, J., and
Han, R. (2015). CRISPR-mediated genome editing restores dystrophin expres-
sion and function in mdx mice. Mol. Ther., in press. Published online October 9,
2015.
http://dx.doi.org/10.1038/mt.2015.192.540
Cell Stem Cell
18
, 533–540, April 7, 2016
ª
2016 Elsevier Inc.