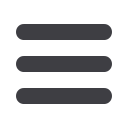
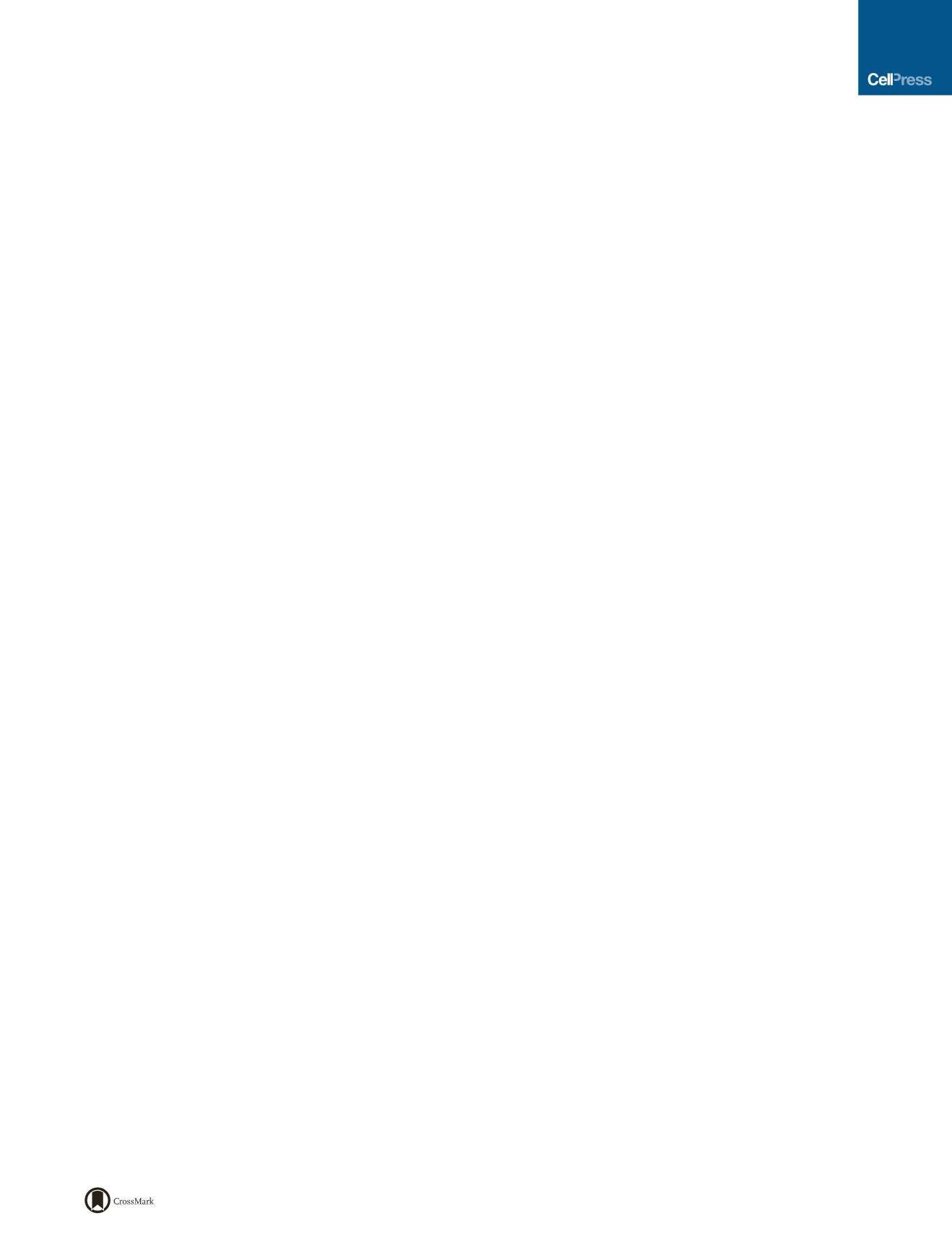
Cell Stem Cell
Resource
CRISPR Interference Efficiently Induces Specific
and Reversible Gene Silencing in Human iPSCs
Mohammad A. Mandegar,
1,
* Nathaniel Huebsch,
1,2
Ekaterina B. Frolov,
1
Edward Shin,
1
Annie Truong,
1
Michael P. Olvera,
1
Amanda H. Chan,
1
Yuichiro Miyaoka,
1,12
Kristin Holmes,
1
C. Ian Spencer,
1
Luke M. Judge,
1,2
David E. Gordon,
3,4,5
Tilde V. Eskildsen,
6,7
Jacqueline E. Villalta,
3,4,8,9
Max A. Horlbeck,
3,4,8,9
Luke A. Gilbert,
3,4,8,9
Nevan J. Krogan,
3,4,5
Søren P. Sheikh,
6,7
Jonathan S. Weissman,
3,4,8,9
Lei S. Qi,
10
Po-Lin So,
1
and Bruce R. Conklin
1,3,4,11,
*
1
Gladstone Institute of Cardiovascular Disease, San Francisco, CA 94158, USA
2
Department of Pediatrics, University of California, San Francisco, San Francisco, CA 94158, USA
3
Department of Cellular and Molecular Pharmacology, University of California, San Francisco, San Francisco, CA 94158, USA
4
California Institute for Quantitative Biosciences, QB3, University of California, San Francisco, San Francisco, CA 94158, USA
5
Gladstone Institute of Virology and Immunology, San Francisco, CA 94158, USA
6
Department of Cardiovascular and Renal Research, University of Southern Denmark, 5000 Odense C, Denmark
7
Department of Clinical Biochemistry and Pharmacology, Odense University Hospital, 5000 Odense C, Denmark
8
Howard Hughes Medical Institute, University of California, San Francisco, San Francisco, CA 94158, USA
9
Center for RNA Systems Biology, University of California, San Francisco, San Francisco, CA 94158, USA
10
Department of Bioengineering, Stanford University, Stanford, CA 94305, USA
11
Department of Medicine and Cellular and Molecular Pharmacology, University of California, San Francisco, San Francisco, CA 94158, USA
12
Present address: Regenerative Medicine Project, Tokyo Metropolitan Institute of Medical Science, Tokyo, 156-8506, Japan
*Correspondence:
mo.mandegar@gladstone.ucsf.edu(M.A.M.),
bconklin@gladstone.ucsf.edu(B.R.C.)
http://dx.doi.org/10.1016/j.stem.2016.01.022SUMMARY
Developing technologies for efficient and scalable
disruption of gene expression will provide power-
ful tools for studying gene function, develop-
mental pathways, and disease mechanisms. Here,
we develop clustered regularly interspaced short
palindromic repeat interference (CRISPRi) to repress
gene expression in human induced pluripotent stem
cells (iPSCs). CRISPRi, in which a doxycycline-induc-
ible deactivated Cas9 is fused to a KRAB repression
domain, can specifically and reversibly inhibit gene
expression in iPSCs and iPSC-derived cardiac pro-
genitors, cardiomyocytes, and T lymphocytes. This
gene repression system is tunable and has the poten-
tial to silence single alleles. Compared with CRISPR
nuclease (CRISPRn), CRISPRi gene repression is
more efficient and homogenous across cell popula-
tions. The CRISPRi system in iPSCs provides a
powerful platform to perform genome-scale screens
in a wide range of iPSC-derived cell types, dissect
developmental pathways, and model disease.
INTRODUCTION
To understand the biological roles of genes in development and
disease, we must decipher the relationships between genotype
and phenotype. Until recently, RNAi has been the most
commonly used loss-of-function tool to study human biology
(Boettcher and McManus, 2015). However, RNAi suffers from
off-target effects and incomplete silencing of the desired gene
(Jackson et al., 2003; Kim et al., 2013b; Krueger et al., 2007).
Alternatively, programmable nucleases, such as zinc-finger
nucleases (ZFNs) and transcription activator-like effector nucle-
ases (TALENs), allow more precise gene editing in model organ-
isms, particularly in mammalian and human systems (Gaj et al.,
2013; Kim and Kim, 2014). While ZFNs and TALENs are efficient
tools for targeting single alleles, they cannot be easily used for
library-scale loss-of-function studies.
In 2012, clustered regularly interspaced short palindromic
repeat (CRISPR) technology emerged as a new tool for gene ed-
iting. This technology is a microbial adaptive-immune system
that uses RNA-guided nucleases to recognize and cleave foreign
genetic elements (Doudna and Charpentier, 2014; Wiedenheft
et al., 2012). The recently engineered CRISPR/Cas9 system con-
sists of two components: a single-chimeric guide RNA (gRNA)
that provides target specificity and a CRISPR-associated protein
(Cas9) that acts as a helicase and a nuclease to unwind and cut
the target DNA (Cong et al., 2013; Mali et al., 2013). In this sys-
tem, the only restriction for targeting a specific locus is the pro-
tospacer adjacent motif (PAM) sequence (‘‘NGG’’ in the case of
Sp
Cas9) (Doudna and Charpentier, 2014).
CRISPR nuclease (CRISPRn) has been used for genome-scale
screens to identify essential genes for cell viability in cancer and
embryonic stem cells (Shalem et al., 2014) and human leukemic
cell lines (Wang et al., 2014, 2015). However, CRISPRn may not
be the most robust system for loss-of-function studies, because
it is limited by the number of cells within a population that do not
produce knockout phenotypes (Gonza´ lez et al., 2014). In addition,
partial loss- or gain-of-function phenotypes can be generated by
Cas9-induced in-frame insertion/deletions (INDELs) and hypo-
morphic alleles (Shi et al., 2015), which can obscure the readout.
The nuclease deactivated version of Cas9 (dCas9) blocks
transcription in prokaryotic and eukaryotic cells (known as
CRISPR interference; CRISPRi) (Qi et al., 2013). More recently,
dCas9 was fused to the Kru¨ ppel-associated box (KRAB)
repression domain to generate dCas9-KRAB, producing a
Cell Stem Cell
18
, 541–553, April 7, 2016
ª
2016 Elsevier Inc.
541